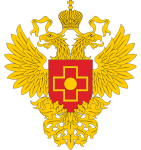
ORIGINAL RESEARCH
Neurophysiological method for studying changes in the brain’s default mode network activity
1 Institute for Physics and Engineering in Biomedicine, National Research Nuclear University MEPhI, Moscow, Russia
2 La-Salute Clinic, Moscow, Russia
Correspondence should be addressed: Sergey A. Gulyaev
Kashirskoe shosse, 31, Moscow, 119604, Russia; ur.xednay@ssurgres
Compliance with ethical standards: the study was approved by the Ethics Committee of the National Research Nuclear University “MEPhI” (protocol № 09-01/23 of 09 January 2023), it was conducted in accordance with the principles of the Declaration of Helsinki issued in 1964 and its subsequent revisions.
Curiosity about the activity of neural networks in the human brain results from the search for definition of human selfconsciousness. As far back as 1637, R. Descartes made the allegation “Je pense, donc je suis” (I think therefore I am) in his treatise “Discours de la méthode” [1]. He defined human selfconsciousness as a key factor inherent only in human being. However, it is generally accepted that organization of thinking is individual in its essence. Social influence may create some similarity of views and systems of cultural perception, however, the thinking process in each individual person appears to be individual and unique when the issue is considered from the perspective of any specific case.
The human brain never loses its activity, even in the resting state. The brain processes the accumulated information that is stored in the memory and makes the goal-directed decisions based on this information. According to the modern literature [2], these processes are strongly associated with the activity of the brain's default mode networks (DMN), the complex neuronal structures showing their activity beyond implementation of the effector neural functions. The mode of activity in such networks depends on numerous factors, such as intensity of recall, analysis of the current situation, planning of processes, etc., however the study of brain activity in different groups of people during transmission of information revealed some similarities of the results [3]. The studies made it possible to detect the characteristic changes in the pathological process intensity resulting from the effects of spontaneous discharges in patients with idiopathic generalized epilepsy and people with autism [4–6]. Alterations of activity in these brain networks associated with various subtypes of depression have been demonstrated [7, 8]. These papers support the idea that the brain function is implemented via activity of separate neural networks or autonomous parts of the common brain network, that are capable of exerting activity not only when the brain is engaged, but also during the periods of relaxed wakefulness, as the components directly related to individual characteristics of the person's thinking.
It is believed that the main DMN of the brain consists of the posterior medial cortex, medial prefrontal cortex, and the temporoparietal junction, thereby integrating the areas responsible for estimation of information and the regions involved in the information analysis [9]. This construct is considered as a kind of “inherent” system showing high activity at rest and decreased activity when engaged in executing experimental tasks. Some researchers compare this phenomenon with the “mind-wandering” associated with activity of the tertiary cortical areas responsible for analysis of the past and modeling of the future [10–12]. Mind-wandering shows up in the form of spontaneous imagery. Furthermore, is has been suggested that the common brain network is divided into networks of the “internal” and “external” systems [13], similar to the functional concept of the “internal” and “external” information processing pathways [14]. However, these assumptions require a more detailed objective confirmation involving further research with the use of more accessible methods than the currently available RS-fMRI technique.
The first objective studies of the brain's DMN activity were conducted using neuroradiological methods, specifically positron emission tomography and single photon emission computed tomography (PET/SPECT). However, such an approach requires administration of radioisotopes to humans that makes it possible to conduct only one-off studies. Today, the RS-fMRI is a leading diagnostic method. This is a more safe method that has been put into practice after the study conducted by Biswal et al. that was focused on assessing the functions of motor cortex and independent sources of spontaneous activity in the brain [15]. Human brain exerts functional activity even at rest. The main postulate of the resting state fMRI (RS-fMRI) is represented by the differences in blood oxygen levels (BOLD) enabling the detection of active areas in the nervous tissue based on the increased oxygen consumption. To date, inclusion of multiple mathematical methods of data analysis in the method has made it possible not only to identify certain areas showing spontaneous brain activity at rest, but also to acquire information about their interplay by developing the concept of integration of brain networks into a single structure, the connectome [16]. Despite all the benefits of the RS-fMRI [17], significant delays occurring during investigation do not allow one to record rapid processes, since the BOLD signal, that is based on transformation of oxyhemoglobin into carboxyhemoglobin and is associated with alterations of the nervous tissue activity, is generated within 2 s at an average. The technique requires using additional equipment and specific software (related to the system for suppression of noise of the equipment running), it also has low temporal resolution that makes it difficult to record rapid changes in brain activity [18–20].
In 2010, the idea of using EEG, the method to record activity of the brain's DMN by assessing certain brain rhythms, was proposed and implemented [21]. However, the study results that reflected the data of frequency analysis made it impossible to judge the possibility of the technique widespread introduction into the diagnostic process.
According to the PubMed database, at least 300 papers on using the frequency analysis of EEG signals for assessment of the brain's DMN had been published over the next 11 years. All the papers describe two common problems: difficulties in matching the results with the RS-fMRI data and low spatial resolution associated with specific pattern of biopotential distribution over the scalp surface [22, 23]. As a result, despite the occasionally emerging interest, neurophysiological methods, even those implemented on the basis of advanced EEG systems, are seldom used to study the structure and functions of distinct brain networks.
However, a specific way to record the activity of distinct groups of neurons by assessing the EEG signal was proposed by D. Lehmann as early as in 1998: after monitoring certain variants of the compound scalp potential he concluded that the compound electrical activity of the brain could be represented by a sequence of bioelectric patterns that were fixed in time and had a duration of about 40–120 ms, within which the distributed pools of neurons showed synchronous activity and generated stable scalp spatial potential topographies, referred to as EEG microstates [24, 25]. It was concluded that the duration of individual microstate could be interpreted as a reflection of preservation and stability of function of the neuronal assembly the microstate was based on, and the recording frequency could be interpreted as activity of certain neural generators during implementation of the studied brain function. However, recording of isolated EEG microstates still not allowed to define brain structures (networks) that were the sources of activity and the extent to which this process was specific for thinking.
In 1994–1997, R.D. Pascual-Marqui proposed the system for the EEG inverse problem solution based on the technique of matching the dipole localization to the layered head model, called low resolution brain electromagnetic tomography (LORETA).
Conclusion about the localization (1998) was made based on the statistical parametric mapping applied to the LORETA scans with high temporal resolution. Starting from 1999, the technique was enhanced by quantitative neuroanatomy based on the templates provided by the Brain Imaging Centre of the Montreal Neurological Institute (MNI). The combination of these solutions brought LORETA to the level of conventional functional imaging methods, such as PET and fMRI [26].
In 2008, the team of R. Grech assessed various techniques of dipole source localization and concluded that sLORETA provides the best solution among the most widely used officially presented and registered EEG post-processing and analysis tools for localization of sources that exert rhythmic activity considering both localization errors and false sources. In 2014, simultaneous fMRI-EEG studies showed that the LORETA technique used to determine EEG power for the alpha, beta, delta and theta-bands in the region of interest revealed a strong link between the spontaneous BOLD - fluctuations in the brain's DMN and various EEG rhythms [27]. This suggests that certain extracted neural nerwork is characterized by specific “electrophysiological signature” created by the combination of brain rhythm serving different putative functions [28].
Thus, today, neural networks of the brain can be studied not only by the conventional RS-fMRI method, but also by combining the methods for extraction of cetrain EEG microstates with the EEG inverse problem solution. Such a combination allows one to study their activity with the delay lower than that of neuroradiological methods. Furthermore, spatial resolution of the combination is enough to enable combining with the results obtained by neuroradioimaging methods that are widely used nowadays.
The study was aimed to demonstrate the possibility of recording activity of the brain's DMN by neurophysiological methods and demonstrate adequacy of the data obtained by the example of changes in the studied characteristics in response to the external stimulus evoking a known response. Null hypothesis of the experiment: if no expected changes in bioelectric activity are recorded after applying specific load, the results obtained when studying the relaxed wakefullness state with the eyes closed do not represent activity in the brain's DMN.
METHODS
The study involved the results of EEG tests of 42 volunteers. Inclusion criteria: no diagnosed neurological or mental disorders as stated in the informed consent. Exclusion criteria: the subject is not a native Russian speaker; left-handedness (even the forced right-handedness); the use of psychoactive substances. The age of subjects in the surveyed group varied between 19–40 years, the average age was 32.37 ± 8 years. All the subjects were right-handed (this was confirmed by using the previously proposed technique [29]) Russian-speakers.
EEG fragments showing background activity were compared in the real time mode in the state of relaxed wakefulness with the eyes closed as previously reported [30]. A 52 channel bioamplifier approved for use in medical institutions with the sampling rate of the analog-to-digital conversion of 500 Hz (Medical Computer Systems; Zelenograd, Russia) and the fragment of recording, when the subject listened to a short story in his/her native (Russian) language (3 min), selected from the full EEG record were used.
EEG was recorded using monopolar montage of electrodes with an average reference electrode. This ensured that the potential differences were equal to the baseline scalp potential at each electrode.
The listening test was selected as a test load due to the possibility of executing the test in the state most close to the baseline state of relaxed wakefulness, which enabled assessment of functional test isolated effects on brain activity. Another reason was a relative knowledge of the human auditory perception system and spatial localization of its major components. The subjects had no preliminary acquaintance with the text to listen to, as well as no additional motivation.
All the tests were performed in the dark and quiet room, however, it was decided not to use a completely soundproofed room due to impossibility to exclude the noise signals of the equipment running and the likelihood of the subject's covert psychogenic response to the isolated room. The latter could cause more severe distortion than the interfering tones and noise with the known characteristics that evoked rhythmic activity over the cortical areas 37 and 47.
The common technological stages of the experiment were as follows. Primary testing of the subject in the relaxed wakefulness state with the eyes closed was performed. The judgement of achieving the relaxed wakefulness state was made based on the stabilization of occipital alpha activity and formation of the clearly defined differences between zones. After the relaxed wakefulness state was achieved, a long epoch was recorded for 3–5 min and saved in a separate data file. The patient's state was assessed after data acquisition. If the differences between zones observed in the EEG recording persisted, the functional load was applied that involved listening for 3–5 min (using the audio recording of speech in native language).
The results of EEG tests were saved in a separate file of the European Data Format (*.edf), allowing for further analysis using the sLORETA software package for EEG signal processing.
Artifacts of the recording were suppressed by the independent component analysis allowing one to separate native EEG data from oscillatory phenomena of a different nature, such as muscle tension, electrooculogram, ECG, etc. After the artifacts were removed from all the selected epochs of the recording, individual stable EEG microstates were calculated by K-means clustering using the software package (sLORETA; Switzerland). It is believed that the minimum number of active neural networks that is enough to describe the activity observed in the relaxed wakefulness state is four [31, 32]. That is why we used a theoretical model consisting of eight contingent neural networks with the twice higher level of base number discretization in accordance with the basic provisions of the Nyquist-Shannon-Kotelnikov sampling theorem to confirm the data obtained and prove the possibility of the brain networks' response to the functional stimulus [33].
Then distinct microstates reflecting the activity of certain groups of neurons [34], that had been extracted from the recording, were subjected to the EEG inverse problem solution in accordance with the previously reported algorithm [35] allowing one to define the main aggregate source of cortical rhythmic activity within Brodmann area 1 (implemented in the sLORETA software package) according to the spatial coordinates stated in the MNI digital MRI atlas [36]. The results obtained were represented as diagrams reflecting the quantitative representation of the EEG microstate recording in accordance with the localization of certain cortical areas.
Thus, the general research model involved extraction of at least eight most frequently repeated sequences associated with the activity of certain cortical macronetworks, that were later spatially localized by using the algorithm for the EEG inverse problem solution, from the general EEG recording. Such an approach allowed us to both define the variants of brain activity alterations and their spatial connection with distinct anatomical structures.
Statistical data processing was performed using the SPSS Statistics 23.0 software package (IBM; USA) in accordance with the guidelines [37]. The null hypothesis was rejected at р < 0.05 by using the chi-squared test.
RESULTS
Testing primary data for consistency using the Cronbach's alpha test showed that the Cronbach's alpha was 0.89, which was typical for high internal consistency of the primary sample and enabled further calculations.
Monitoring of bioelectric activity in the relaxed wakefulness state showed that the largest number of rhythmic phenomena occurred in the occipital regions (Brodmann areas 17, 18, 19). This reflected rhythmic activity of cortical structures observed in the relaxed wakefulness state, which was well-known to the specialists. Such activity was represented by alpha rhythm produced by visual cortical areas in the “standby” or “rest (idle)” mode [38–39].
The rhythmic activity recorded over the cortical auditory and language areas was confined to the areas 22, 37 and 40, which are principally responsible for perception of musical tones rather than language components that are characterized by the involvement of the Wernicke's area tertiary cortical areas (areas 39 and 40). The involvement of sensorimotor cortical areas (1 and 2) was also reported (only within a single microstate) together with rhythmic activity over the supplementary motor area (area 6), tertiary cortical areas of the area 7 and prefrontal cortical area (area 10) that charactesized external information processing (fig. 1).
When executing the functional test that involved listening we expected to detect the activity over the cortical areas related to realization of speech function: areas 37–47 (responsible for musical perception and production of music), area 22 (responsible for perception of tones and noise), areas 39, 40 (constitute tertiary cortical areas of the Wernicke's area), and areas 44, 45 (constitute teriary Broca's area).
Individual analysis of each and every case revealed various individual sequences of the involvement of certain cortical areas that formed the unique profile of each subject. It was also noted that the sequences not necessarily included the well-known speech areas.
In fact, several variants of the response represented by sequences showing involvement of noise perception (area 22) together with area 39, the combination of rhythmic activity over the areas 37 and 47 responsible for musical perception and production of music, conventional involvement of the auditory and language areas 39, 40, 44, and 45, and the variants not invoving the areas related to auditory and language perception were recorded.
However, the group comparison revealed the phenomenon of ordering the ordered structure of EEG microstates compared to the relaxed wakefulness state with no load applied and the changes in characteristics of rhythmic activity exerted by the cortical structures (fig. 2).
Thus, despite the persisting predominance of rhythmic activity recorded from the areas 17, 18, and 19 that reflected the subjects' staying with their eyes closed, activity of the cortical areas responsible for information processing was concentrated in the cortical areas 6, 7, and 10. Furthermore, in addition to activity recorded from the areas 22, 37, and 40, the emergence of rhythmic activity over the areas 39, 44, 45, 46, and 47 associated with the structures constituting the lateral stream of the sensory system involved in speech (Wernicke's, Broca's areas) was detected in patients during listening.
Statistical comparison using the nonparametric chi-squared test made it possible to define a satisfactory significance level for the data obtained (< 0.001), thereby allowing us to reject the null-hypothesis of the study.
DISCUSSION
Currently, the methods of assessing the brain's DMN reported in the majority of scientific papers are implemented using the direct results of EEG frequency analysis [21–23]. Matching the data obtained using EEG and the resting state fMRI is one of the challenges faced by the researchers, since the technique for extraction of the DMN activity during fMRI involves representation of the functionally active areas of cerebral structures as the zones showing alterations in BOLD signal, while EEG analysis detects rhythmic activity, that does not represent a physiological equivalent of excitation, in the area of interest [35–39].
According to our findings, the simplest solution of this problem may be recording the prolonged sequence of phenomena of the transition from excitation to the state of generating rhythmic activity within the prolonged epoch of the recording integrated into a single EEG microstate.
In this study we used a proposition that the recording of distinct EEG microstates reflects the activity of the finite number of neural networks, that is why applying the technique of the EEG inverse problem solution to each extracted EEG microstate makes it possible to determine spatial distribution of certain brain structures involved in realization of total brain activity at rest with higher spatial resolution than the use of frequency analysis only.
In particular, it was shown that the activity of cortical structures, including sensorimotor and visual cortex, was determined in the state of relaxed wakefulness, which, in our opinion, could be characteristic of brain activity related to analysis and processing of information the human deals with at rest [10, 13].
At the same time, inclusion of the functional stimulus that resulted in the significantly changed activity and the expected involvement of the tertiary auditory areas allowed us to interpret the data obtained by analysis of the relaxed wakefulness state as a true manifestation of activity exerted by the brain's resting state networks.
Thus, introduction of the model of EEG microstates shaped the native EEG signal pre-processing, thereby allowing us to extract the signal components produced by certain neural structures. The combination of the model with solving the inverse problem implemented in the processing software packages that are available for researchers makes it possible to propose a simple and more cost-effective technique to determine alterations in neuropsychological processes.
CONCLUSIONS
The study demonstrates the possibility of performing EEG analysis of the brain's DMN and opens up the prospect of performing affordable and objective studies of cognitive processes to obtain the results with high temporal resolution, since the process of the brain's bioelectric activity recording is not limited to slow biochemical reactions. Such an approach will make it possible to assess spatial localization of the brain structures responsible for implementation of distinct cognitive functions and to determine the dynamics and sequence of their involvement in the cognitive process. Such systems can be developed using advanced equipment, including free open source tools. This makes it possible to propose a new approach to extensive development and the use of EEG technology in both scientific research and practical studies. Active introduction of the systems will allow us to define new prospects in studying human thinking and, perhaps, to propose new cognitive control systems to be used under ordinary conditions and in the environment associated with increased psychoemotional stress.