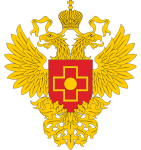
REVIEW
Sars-CoV-2 in the context of coronaviruses and animal models of COVID-19
Federal Research and Clinical Center of Physical-Chemical Medicine of Federal Medical Biological Agency, Moscow, Russia
Correspondence should be addressed: Konstantin S. Gorbunov
Malaya Pirogovskaya, 1a, Moscow, 119435; gro.mcpcr@vonubrog.nitnatsnok
Author contribution: Korenkova AA — data collection, manuscript preparation; Bahmetjev VV — data collection, manuscript preparation; Gorbunov KS — supervision.
SARS-CoV-2 is one of the 3 coronaviruses to have caused an epidemic among humans in the 21st century. Notably, all of those 3 viruses were zoonotic [1]. This underscores the dangers of zoonotic infections to humankind. Coronaviruses have a capacity for recombination and therefore can infect different species. This review looks at SARS-CoV-2 in the context of other coronaviruses that pose a threat to mammals in general and humans in particular. Similar to the studies of SARS-CoV and MERS-CoV, veterinary research of animal coronaviruses, including FCoV (feline infectious peritonitis), CCoV (canine viral enteritis), SADS-CoV (swine acute diarrhea syndrome) and some others, can yield invaluable data for countering SARS-CoV-2.
Developing effective and convenient experimental models of COVID-19 is a pressing concern because animal models are indispensable for studying the pathogenesis of the disease and testing candidate drugs and vaccines.
General characteristics of SARS-COV-2
An RNA virus that caused the pandemic of 2020 and was termed 2019-nCoV or SARS-CoV-2 is a member of the Coronaviridae family. Today, 7 Coronaviridae viruses are known to infect humans; of them 3 are associated with acute respiratory syndromes (SARS-CoV, MERS-CoV and SARS-CoV-2) and 4 (HCoVs) cause only mild respiratory symptoms (tab. 1). According to international reports, all the 4 HCoVs circulate in the human population all year round and are characterized by seasonal incidence peaks [2, 3].
SARS-CoV-2 causes an often asymptomatic disease called COVID-19 [4]. The signs and symptoms observed in patients with mild or moderate COVID-19 remind those of acute respiratory infections and seasonal flu, hampering the diagnosis. Severe COVID-19 can lead to complications, including acute respiratory distress syndrome and multisystem disorders.
The primary route of SARS-CoV-2 transmission is through droplets produced by an infected individual during coughing, sneezing, talking or breathing. This mode of transmission is typically seen in humans and between humans and domestic animals. The novel coronavirus can also spread through fomites, which are objects and surfaces contaminated with biological fluids of infected patients containing viable SARS-CoV-2 virions [5]. It is reported that the pathogen has been detected in wastewater, so it is possible that exposure to contaminated wastewater may result in SARS-CoV-2 infection [6]. Additionally, SARS-CoV-2 RNA has been detected in blood, mucus, saliva, urine, feces [5] and sperm [:lit_7. The definitive factor ensuring the spread of the virus through direct contact is its viability outside the host. According to the literature, the reported viability of the novel coronavirus varies from a few hours to a few weeks, depending on the type of contaminated surface and some environmental factors.
The mechanism used by SARS-CoV-2 to invade the host cell is still debatable. There are a few possible entry points, including 2 cell receptors CD147 [8] and GRP78 [9]; however, the dominant cell entry mechanism is through the membrane receptor ACE2 [10]. The S-protein, which forms spikes on the surface of the viral nucleocapsid, anchors to ACE2, and the subsequent cell entry is mediated by the transmembrane serine protease 2 (TMPRSS2) [11]. A similar mechanism is employed by SARS-CoV [12].
ACE2 is expressed in more than 150 different cell types found in almost all human tissues and organs [13], but its expression levels vary depending on the cell type. ACE2 is present on the membranes of type II pneumocytes, small intestine enterocytes, endothelial cells of arteries and veins, and smooth muscle cells of most human organs. Given that SARS-CoV-2 uses ACE2 to enter the cell, one can expect that some signs of COVID-19 will be observed in ACE2-expressing tissue.
Evolution of SARS-COV-2
It is reported that HCoVs are descended from animal coronaviruses. For example, SARS-CoV, MERS-CoV, HCoV-NL63 and HCoV-229E are related to bat coronaviruses, whereas HCoV-OC43 and HKU1, to rodent coronaviruses [1]. SARS-CoV-2 is likely the product of genetic recombination that occurred in a natural reservoir, the Chinese population of bats [14]. The SARS-CoV-2 genome shares 89% sequence homology with SARS-like-CoVZXC21 and 96% sequence homology with RaTG13.
Being an RNA virus, SARS-CoV-2 has 2 evolutionary strategies: 1) genetic drift or natural selection of mutations and 2) exchange of genetic material with other viruses through recombination [15].
Between December 2019 and September 2020, over 18,500 SARS-CoV-2 genomes were sequenced. Based on the sequencing data, it was concluded that the novel coronavirus is relatively conserved. This means that future vaccines against SARS-CoV-2 might be equally effective against any of its variants [16]. Most mutations detected in SARS-CoV-2 genome do not affect the properties of the pathogen or reduce its pathogenicity/virulence toward humans. The rate of such mutations, including D614G, can be explained by the founder effect.
Structurally, SARS-CoV-2 is a spherical or pleomorphic enveloped particle containing single-stranded positive-sense RNA. SARS-CoV-2 RNA is complexed with the nucleoprotein inside the viral capsid formed by the matrix protein [17]. The club-shaped spikes of the glycoprotein known as the S protein protrude from the viral envelope. The S protein binds to the membrane of the host cell and mediates the invasion.
Numerous animal studies have demonstrated that coronaviruses frequently undergo genetic recombination. For example, S-protein recombination seems to be a common event in feline and canine coronaviruses [18]. The S-protein is a membrane glycoprotein composed of 2 subunits: S1 and S2. The S1 subunit enables the virion to latch onto the host cell exploiting the interactions between its receptor-binding domain (RBD) and the receptor located on the surface of the host cell. The RBD-encoding gene region is the most variable part of the coronavirus genome. The genetic flexibility of the S protein and especially its RBD might allow the pathogen to less specifically bind to ACE2 receptors in a variety of animal species and thus expand the range of possible hosts [14]. Mutations in the S protein might induce conformational changes, which, in turn, affects viral antigenicity. So far, a few mutations have been discovered in the S1 receptor binding region but they have not undermined the ability of the virus to bind to ACE2 in humans, pigs, civets, and bats [19]. Recombination between gene regions coding for the S1 and S2 subunits of the S protein was deemed as one of the major mechanisms facilitating the emergence of human SARS-CoV strains from bat and civet ancestors [20].
Because SARS-CoV-2 is transmitted more rapidly that it evolves, its population is becoming more homogenous, with a median of 7 nucleotide substitutions between genomes. There is evidence of purifying selection, but little data is available to suggest diversifying selection; the rates of nucleotide substitutions are comparable between structural and non-structural genes [16]. Most mutations acquired by the virus are phenotypic and thus provide information on the geographic and population origin of the viral lineage.
The S protein of SARS-CoV-2 effectively binds to ACE2 receptors in humans, ferrets, cats and other mammals sharing high receptor homology [21]. The remarkable diversity of species susceptible to SARS-CoV-2 suggests that the pathogen can cross the species barrier and encounter other coronaviruses, which might result in a recombination event and thus give birth to novel viral strains and species. In the past 20 years, 3 coronaviruses have spilled over from zoonotic reservoirs; this underscores the need for surveillance of animal coronaviruses [22], the importance of studying mutations that allow zoonotic viruses to perform a host jump and the usefulness of medical zoology research.
Recombination events among HCoVs have been amply described in the literature [23]. For example, the screening of specimens obtained from Kenyan bats allowed researchers to identify a few viruses exhibiting genetic similarity to HCoV-NL63 and HCoV-229. These viruses were reported to have an eventful history of genetic recombination, including 2 interspecies recombination events involving the S-protein gene. This suggests that the S-protein gene might be a recombination hot spot in coronavirus genomes [24].
Animal coronaviruses are a potential threat to humanity
Many mammalian coronaviruses have been well studied and characterized by veterinarian scientists. For example, it is known that β-coronaviruses encompass human viruses HCoV-OC43 and HCoV-HKU1 that cause acute respiratory infections in humans and a number of other viruses that infect dogs, cats, cattle, pigs, horses and camels. HCoV-OC43 and bovine BCoV share 95% sequence homology, whereas SARS-CoV-2 shares almost 96% sequence homology with RaTG13 (member of the SARSr-CoV group) isolated from the horseshoe bat (Rhinolophus affinis). Viruses genetically close to SARS-CoV-2 have been isolated from other bats and palm civets (Nandinia binotata) [15]. However, although SARS-CoV-2 and bat CoV RaTG13 share almost 98% homology in the sequences coding for the S protein, the SARS-CoV-2 genome contains an insertion of a furin cleavage site (RRAR) in the S1/S2 region. This multibasic cleavage site might be associated with the high virulence of the novel coronavirus [19]. A virus related to SARS-CoV-2 has been isolated from pangolins (Manis javanica), which is why these animals were thought to be an intermediate host for SARS-CoV-2 [25]. Animal hosts of β-coronaviruses are potential models of infectious disease caused by this group of viruses, including SARS-CoV-2. Notably, over time intermediate hosts can become natural reservoirs for coronaviruses, whereas viruses predominantly harbored by intermediate hosts can accumulate mutations independently. Besides, in the intermediate host the virus can accumulate mutations allowing it to successfully invade the final host. If the natural host is infected by different populations of the same viral species, recombination between these populations will drive the emergence of new strains [20].
Bats harbor a greater diversity of zoonotic viruses than other mammals [26]. The list of viruses hosted by bats includes relatives of SARS-CoV, MERS-CoV, HCoV-229E, HCoV-NL63 [27], and SARS-CoV-2 [25]. The fact that bats are lowly susceptible to infectious pathology caused by the viruses they host requires thorough investigation. But reports of coronaviruses crossing the species barrier [1] raise the need for close wildlife disease surveillance and research into the potential routes of viral transmission between species, because each host jump increases the odds of a fundamentally new recombination event associated with the virome of the host.
A host can be simultaneously infected with several coronaviruses, which creates favorable conditions for recombination and affects the clinical picture. Coinfections aggravate the course of a primary disease. In human hosts, SARS-CoV-2 can cooccur with other viruses, including coronaviruses that cause respiratory infections [28]. The most common SARS-CoV-2 coinfection is influenza A virus. Respiratory coinfections are negatively correlated with the accuracy of COVID-19 diagnosis, and clinical manifestations of COVID-19 do not always raise suspicions about the presence of another respiratory (viral, bacterial or fungal) pathogen, which may result in the wrong treatment choice. Unfortunately, coinfections in patients with COVID-19 remain heavily understudied [29]. Coinfection can contribute to the mutability of the coronavirus. Coronaviruses coexisting in one host undergo frequent recombination events and mutate actively [24]. So far, of 39 currently known coronaviruses [30] 7 are capable of infecting humans. These viruses pose a threat to agriculture and human health. Identifying the reservoirs of zoonotic pathogens is crucial to effective disease control and prevention [25].
Animal models of COVID-19
Animal models are indispensable for conducting preclinical trials of candidate drugs and vaccines and studying the pathogenesis of SARS-CoV-2 infection. Since the clinical manifestations of COVID-19 differ significantly among the infected individuals, it is important to create models reflecting different degrees of disease severity. This will allow researchers to preclinically assess the efficacy of candidate drugs depending on the severity of the disease. Studying the diversity of species that host the virus in question might help to find a suitable animal model. Animals in which the virus replicates but does not cause overt pathology are reservoirs for the infection; their surveillance is critical for preventing the outbreaks of the infection. Animals that can transfer the virus on their skin or fur constitute a separate category. For example, SARS-CoV-2 RNA has been detected in the biological samples of domestic dogs and cats, tigers and lions [15]. However, a positive PCR test does not prove that the tested animal is sick or is the carrier of viable virions. Nevertheless, the fact that the virus can be transmitted from humans to domestic animals is a worrying sign [15], although there were no reports of animal to human transmission.
Initially, the search for animal models of COVID-19 focused on the animals that had been previously regarded as candidate models for SARS and MERS. Unfortunately, none of them were fairly suitable to study these two viruses [31].
Attempts were made to study SARS-CoV replication in Syrian and Chinese hamsters, civets and non-human primates (NHP), such as rhesus monkeys, crab-eating macaques, African green monkeys, etc. [32]. Mice and ferrets were more susceptible to SARS-CoV infection but resistant to MERS-CoV, due to the properties of their DPP4 receptors [25]. Rabbits were not investigated as a potential model of SARS-CoV [31] and turned out to be an unsuitable research model for MERS [33]. A study demonstrated that ferrets (Mustela furo) and domestic cats (Felis domesticus) were susceptible to SARS-CoV and could effectively spread the virus to other noninfected animals they were housed with [34]. Likewise, domestic cats and ferrets can be infected with and spread SARS-CoV-2 [35], which makes them a promising SARS-CoV-2 candidate model.
American mink (Neovison vison) bred on fur farms are susceptible to SARS-CoV-2, which they presumably contracted from humans [36]. Thus, mink can be a good animal model for studying COVID-19 and other coronaviruses capable of binding to ACE2. Advantageously, there are well-established housing and care protocols for mink and ferrets. Mink can be used to model severe and moderate COVID-19. However, there are still a few issues related to the housing of these animals in a laboratory environment [37].
Tigers and lions have been reported to develop COVID-19 symptoms [15]. The fact that two distant families of the mammalian order Carnivora, Mustelidae and Felidae, can so easily contract the virus and develop COVID-19 indicates that the wide variety of animal species can act as a reservoir for SARS-CoV-2 [34]. It is possible that some of them might become a new effective model for COVID-19. More different mammals need to be investigated in order to identify new potential sources of the infection and find suitable research models. tab. 2 describes a few animal models for SARS-CoV-2.
Northern treeshrews (Tupaia belangeri chinensis) and Egyptian fruit bats (Rousettus aegyptiacus) were also investigated as candidate models of SARS-CoV-2 but they did not develop any pathology following a challenge with the coronavirus, although the virus was detected in the multiple organs of these animals [25]. Therefore, the northern treeshrew and the Egyptian fruit bat do not hold promise as COVID-19 models.
The susceptibility of nontransgenic mice to the coronavirus can be significantly affected by their genetic traits unrelated to ACE2 [25], which may skew the clinical picture in a way that cannot be predicted.
Alpacas (Vicugna pacos) and dromedary camels (Camelus dromedarius) were used as the first MERS models [33, 43]. But because the upkeep of dromedary camels is quite challenging and these animals can pass the infection to humans, researchers had to give up the idea of using them as a MERS model. Alpacas infected with MERS-CoV remained clinically healthy although they did produce antibodies [5]. Since there were more convenient animal models, the use of tylopods for studying SARS-CoV-2 was eventually discontinued.
Syrian hamsters turned out to be the most effective and cheap model of COVID-19. Cats and ferrets might hold some promise but their upkeep is more difficult. Despite the absence of data, mink are considered to be a promising model for SARS and MERS. NHP models are vigorously used in preclinical trials of candidate drugs and vaccines against COVID-19.
CONCLUSIONS
The diversity of coronaviruses poses a serious threat to epidemiologic safety. Future pandemics can be prevented using an integrated approach to medical, veterinarian and zoological studies. In the 20th century, the effective surveillance of zoonotic infections contained the spread of zoonotic viruses from wildlife to humans. Knowing the routes of viral transmission is as important as understanding the coevolution of the virus and its host. Studies of animal coronaviruses might provide invaluable data that will serve as a starting point in researching SARS-CoV-2 and other human coronaviruses. Animal models are useful in modeling human diseases, studying the progression of the disease and exploring the properties of the virus. Expanding the range of model animals will allow us to find the optimal models for studying the pathogenesis of COVID-19 and testing candidate drugs and broaden our research potential needed to counter new infections in the future.
Using a systemic biological approach to the analysis of viral diversity and the reconstruction of the interactions between the virus and its host under all possible outcomes, we will be able to effectively contain potential threats and prepare for new pandemics. The human body is an ecosystem, so studies looking into the interactions between the virus and its human host should take into account the interactions between the virus and the human microbiota, as well as the probability of recombination with viruses constituting the human virome. Humans are part of terrestrial ecosystems, so it is important to trace the transmission of the virus from animals to humans and from humans to animals as new mutant viral strains can be passed back from its new host to the human population.