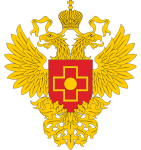
This article is an open access article distributed under the terms and conditions of the Creative Commons Attribution license (CC BY).
REVIEW
Brain concussion in young athletes: major pain points
1 Federal Research and Clinical Center for Sports Medicine and Rehabilitation of the Federal Medical Biological Agency, Moscow, Russia
2 Pirogov Russian National Research Medical University, Moscow, Russia
Correspondence should be addressed: Maria S. Tarasova
Raevskogo, 4, 121151, Moscow; ur.abmftrops@smavosarat
Funding: this review is part of the applied research study on the Clinical and prognostic significance of neurometabolic cascade for the development of delayed complications of hypoxic and traumatic brain injuries in athletes (Neurocascade-20, ID 76.35.41) conducted by the Federal Research and Clinical Center of Sports Medicine and Rehabilitation of FMBA under the State Assignment for years 2020–2021.
Author contribution: Klyuchnikov SO searched and analyzed the literature and contributed to writing the manuscript; Feshchenko VS contributed to writing the manuscript and edited the final version; Zholinsky AV edited the final version of the manuscript; Tarasova MS, Slivin AV, Efimov PV searched and analyzed the literature.
Numerous studies conducted in recent decades and the debate they sparked in top-tier scientific journals and at international forums have generated vast amounts of data on sport-related concussions (SRCs). A series of systematic reviews and metaanalyses have described how SRC characteristics vary depending on the type of sport, duration of training activities and sex. A wealth of diagnostic modalities, protocols, policies and laws have been elaborated to manage SRCs in a clinical setting [1, 2]. One would think that the amount of knowledge accrued to date and the advances in medical and biological technology would be enough to solve SRC-associated diagnostic and therapeutic challenges. Yet many of the complex aspects of SRCs remain unresolved. Paradoxically, there is no clear-cut definition for this injury type, no objective diagnostic criteria and consensus management strategies that would account for the young athlete’s age.
Very mild symptoms, the lack of specific presenting complaints and a relatively short recovery period in the absence of medication therapy foster a misconception about the insignificant impact of brain concussion on the young athlete’s health. There is convincing evidence accumulated to date that even a single SRC may adversely affect academic achievement; repeated SRCs dramatically increase the risk of academic performance decline [3]. Children are at particularly high risk for long-term sequelae after SRC, especially if another episode of brain concussion occurs during the recovery period. [4; 5]. Sports medicine physicians are faced with a multitude of SRC-related questions that need to be addressed.
One of them is the incidence of brain concussions. SRC statistics cited in the literature traditionally refers to the American population of young athletes, largely due to the abundance of data generated from large sample sizes; in the USA, there are 1.1 to 1.9 million SRC reported cases among children annually [6]. According to other sources, the annual incidence of SRC among athletes varies from 1.6 to 3.8 million a year, being the second common cause of head injury after car accidents [7]. These statistics include college and school athletes and recreational injuries sustained during recreational activities (as opposed to practice and competition). Recent epidemiology studies have reported a surge in SRC incidence. In 2006, there were 569.4 SRCs per 100,000 injuries; in 2012 their number increased to 807.9 per 100,000 injuries [8]. This increase may be explained by but is not limited to the growing attention to SRC from doctors, coaches and parents [9]. Some amendments to legislation and socioeconomic factors discussed in [10; 11] may, too, have contributed to the active reporting of SRCs.
Although it is well known that about 70% of injuries are sustained by children under 19 years [12], until recently pediatric and adolescent SRCs were considered a minor injury. It was argued that SRCs are well compensated by physiological and adaptive resources, and their long-term effects are rare and/ or mild [13]. The argumentation was based on multiple clinical studies which reported obvious clinical symptoms or signs of neuropsychological disturbance after SRC in only a small proportion of the affected children [14].
Innovative diagnostic approaches, experimental, clinical, functional and neurophysiological studies of the brain increasingly suggest that conventional methods of clinical assessment are insufficient to detect and differentiate between subtle and heterogenous pathologies associated with SRC, especially in children. There is mounting evidence that SRC may have long-term effects on pediatric and adolescent health that persist past recovery. Most clinicians use commercial tests like ImPACT or SCAT for the clinical evaluation of SRC. Indeed, these pediatric tests have a number of indisputable advantages over baseline concussion tests, but studies have demonstrated their insufficient reliability and sensitivity for detecting SRC and predicting its sequelae that develop after the acute phase of the injury [1; 15; 16].
Many researchers have convincingly demonstrated that most SRCs sustained by athletes of any age are not reported to healthcare providers. There are a few major reasons for that. Coaches do not always pay careful attention to the symptoms experienced by players; athletes do not know much about this type of injury or tend to underreport any health-related issues out of fear of being suspended from practice or competitions, etc. According to [17], 40 to 76% of young athletes attempt to conceal their injuries. According to [18], up to 80% of adolescent rugby players do not report their injuries or return to active training before making a complete recovery.
The incidence of SRC depends on the type of sports. The most injurious sport is American football. Lacrosse, ice hockey, martial arts, snowboarding and some other kinds of sports, including contact sports, are associated with high risk of SRC [5].
Pathophysiologically, SRCs is characterized by temporary neuronal and axonal dysfunction and impairments of the vascular network of the brain due to a cerebrospinal fluid shock wave and rotational mechanism; this impacts neurotransmission and neurometabolism. Besides, SRC can provoke a petechial hemorrhage around the Sylvian aqueduct [19]. Recent studies conducted in different parts of the world have paved the way to understanding fundamental SRC-inducing biokinetic mechanisms. The most crucial of them are head accelerations and decelerations, which usually alternate rapidly during the impact, and head rotation [20]. SRCs are thought to occur due to a direct blow/impact to the head. Importantly, SCRs can be caused by blows to other parts of the body if the applied force is transmitted to the head [1]. Weak neck muscles typically seen in women and children might be critical for a concussion. Some authors think that children are at greater risk for SRC because of weak neck muscles as they cannot dampen the impact to the head [21].
Pediatricians have long known that vigorous accelerationdeceleration of the head in children under 5 years often has dramatic consequences and provokes the so-called shaken baby syndrome. The term whiplash shaken infant syndrome (WSIS) was coined to describe a diagnostically important combination of symptoms, including retinal hemorrhage, subdural and/or subarachnoid hemorrhage with or without mild injury to the skull [22].
Unlike older children, the brain of younger children has a number of physiological characteristics predisposing to a dramatic clinical picture after SRC. A big head and weak neck muscles render a child vulnerable to the impact of acceleration and deceleration forces during SRC. Incomplete myelination and highly hydrophilic brain tissue typical for young age also significantly increase the risk of SRC during rapid or sudden head acceleration, deceleration and rotation [23].
Damage to the myelin sheath impairs transmission of nerve impulses and makes the brain more susceptible to injury during repeated impacts. This fact has been proved for hypoxicischemic brain injury and, more specifically, stroke in adult patients. There are ample reports that a past history of brain concussion increases the risk of a repeated concussion 2-5.8-fold [24; 25]. According to experts, repeated SRCs significantly contribute to the development of delayed complications and shape the pattern of recovery [5].
Being a highly heterogeneous group of pathologies, brain concussions are considered minor traumatic brain injuries. The underlying pathophysiology and clinical manifestations suggest that every SRC is unique and is characterized by a wide palette of symptoms ranging in severity. The diversity of SRC manifestations, which often overlap with the symptoms of other conditions, poses a diagnostic difficulty. Neuroimaging techniques (MRI, CT) used in clinical practice do not detect any pathological transformations in the brain after a concussion. However, these techniques help to differentiate SRC from other conditions with similar presentations.
Recent decades have witnessed multiple attempts to create a detailed description and a classification of SRC symptoms in different age groups of athletes involved in various sports. All SRC symptoms can be categorized into somatic, behavioral and cognitive.
The list of somatic SRC symptoms includes headache, nausea, vomiting, blurred vision, eye floaters, balance problems, increased sensitivity to light or noise, and tinnitus.
Among the behavioral and emotional effects of SRC are sleep disturbances, sleepiness, fatigue, irritability, anxiety, and depression.
The group of cognitive symptoms comprises difficulty concentrating or remembering things, slow reaction time, etc. [26].
A systematic review has analyzed the typical signs of SRC described in the literature [2]. The following manifestations were identified as common for athletes:
- observed and documented disorientation/confusion immediately after injury;
- inability to maintain balance within 24 h after injury;
- slow reaction time within 2 days after injury and/or – impaired verbal learning and memory within 2 days after injury.
Owing to the technological advancements in neuroimaging, it is now possible to objectively assess the effects of injury on the brain. According to the currently held view, the definition of brain concussion should be limited to conditions caused by biomechanical exposure and not accompanied by structural changes in the brain. However, there are published diffusion tensor imaging data demonstrating the presence of microstructural brain pathology (slightly disrupted integrity of long white matter tracts, diapedesis hemorrhage) even after minor TBI and suspected SRC. It is hypothesized that such microchanges may be a sign of minimal TBI, but their clinical significance remains unclear.
Nevertheless, research into functional connectivity of the brain suggests that SRC and subconcussive impacts exert a negative effect on neuronal communication in young athletes. For example, a diffusion tensor imaging study [27] revealed that whole-brain fractional anisotropy was significantly increased in adolescent athletes 6 months after brain concussion, suggesting changes to myelination and fiber density [28]. At the same time, despite the disrupted integrity of the white matter inferred from the level of fractional anisotropy in the acute injury phase, children were able to recover the normal values of the measured parameters 6 months after injury [29]. This discrepancy complicates clinical interpretation, dictating the need for further research.
Some valuable data have been generated by neurometabolic studies. Adult athletes with SRC are reported to have elevated glutamate [30], choline [31], creatinine [32] and low N-acetylaspartate [32]. These compounds are important participants of brain metabolism; the levels of their expression correlate with neuropsychological performance [33] and results of experimental tests conducted among athletes [31]. Studies looking into the neurometabolism of children with SRC are scarce. One of them describes age-dependent changes in the metabolic profiles of children [34]. PET studies have confirmed considerable functional shifts in brain metabolism among children with sustained SRC. For example, initially excessive consumption of glucose after injury is followed by an “energy crisis” [35].
The growing interest in brain metabolism is predicated on the idea of using biomarkers in the diagnostics of brain disorders. This idea is supported by compelling scientific evidence and the potential of diagnostic agents demonstrated by stroke studies. There are promising data on the role of neuromarkers in TBI. For example, it has been established that impulse transmission between neurons is driven by changes in the transmembrane potential regulated by ion channels and glutamate receptors. The latter participate in most excitation pathways of the brain and play the key role in neuron plasticity, adaptation, learning, and memory [36].
During the acute TBI phase or axonal injury, massive amounts of glutamate are released into the synaptic cleft, initiating the activation of AMPA receptors that mediate excitation. The N-terminal domain of AMPAR gets cleaved by extracellular proteases, permeates the disrupted blood-brain barrier and enters the bloodstream. The product of AMPAR degradation, the AMPA-peptide, can be detected in the blood. Blood levels of AMPA-peptide were measured in 84 American football athletes as part of a complex diagnostic procedure which included neurocognitive testing and neuroimaging. This study conducted in the USA demonstrated that subjects with SRC had reduced visual memory, low ImPACT scores and elevated levels of the AMPA-peptide. During a 1.5-year-long follow-up, only 18 of 33 athletes recovered normal levels of the peptide and were able to resume active training [37].
In addition to glutamate-associated markers, some other compounds have been proposed for the assessment of brain concussions: S100, glial fibrillary acidic protein, neurotrophic factors, creatinine kinases, etc. However, their practical value as diagnostic markers of SRC in young athletes has not been confirmed. Objective diagnostic criteria for concussions and health assessment criteria for athletes undergoing rehabilitation are yet to be found.
One of the hypothesized pathophysiological mechanisms of SRC is a cerebrovascular reactivity disturbance. It is reported that 16 to 22-year-old athletes with SRC recover from hypo- and hyperventilation more slowly than their healthy peers [38]. SRC may cause chronic cerebrovascular dysfunction. A study describes a significant reduction in cerebral blood flow a month after injury in 11 to 15-year-old children [39]. Their behavioral symptoms completely resolved 2 weeks after injury, but cerebral blood flow disturbances persisted in 64% of the participants. Thirty days after injury, all of the examined children performed well in neuropsychological tests, but 36% of them still had cerebrovascular dysfunction. Pronounced cerebrovascular dysfunction can persist for up to 12 months after injury in athletes who show no clinical symptoms of concussion and perform well in the offered tests [40]. This suggests formation of a long physiologically vulnerable period after SRC when a recurrent event, which does not have to be a biomechanical impact, can increase the risk of developing long-term adverse effects [1].
Research into neuroelectric function of the brain holds promise for shedding light on SRC-induced neurophysiological changes. The highest specificity and sensitivity are demonstrated by the event-related potentials technique. Children with brain concussion exhibit deficits in attention and executive control and are less aware of mistakes they make [41]. Even a single concussion can provoke profound changes in neuroelectric brain function, causing attention deficit, especially in cognitively demanding circumstances [42].
Summing up the above facts, SRC can provoke serious neurophysiological changes in children that can be detected by modern diagnostic tools; however, the neuroanatomic consequences of SRC remain unstudied. Despite some morphological and functional differences, the pathophysiological picture of SRC in young as well as adult athletes is quite distinct and manifests as persistent changes to functional connectivity, cerebral blood flow and neuroelectric function of the brain.
Age is a significant factor for SRC outcomes A series of studies have shown that children recover at slower pace than adults [43; 44]. Longitudinal clinical studies of SRC, which generally report optimistic outcomes, suggest that children under 10 years of age are at significantly greater risk of delayed SRC complications [14; 45]. Extensive research emphasizes the importance of recognizing prepubertal children (under 10 years) as a separate age group that is at high risk of persistent neuropsychological and neuropsychiatric impairments. But careful attention should be paid to young athletes’ health throughout puberty because adolescent athletes recover at slower pace than adults [46; 47].
There is an opinion that this vulnerability of young athletes is largely determined by some age-related aspects of myelination and development of functional connectivity in maturing and developing frontal regions of the young brain [48; 49]. However, it is not shared by everyone in the scientific community. A team of 11 experts led by F. P. Rivara has analyzed a vast array of publications released in 1980–2018 and concluded that there is no convincing evidence that young athletes are more susceptible to SRC than adults [5]. At the same time, the experts are unanimous in stating that the quality of research studies has changed significantly over the past years, which complicates comparison and interpretation of data yielded by of research studies from different time periods.
Traditionally, the contribution of sex differences to SRC outcomes remains the subject of heated debate. Some authors point to the higher incidence of SRC in young female students [50; 51]; these findings are supported by the conclusion of the expert panel [5]. According to the literature, the frequency and intensity of clinical symptoms and the rate of unfavorable outcomes are generally higher for female underage athletes than for young male athletes [52; 53]. This could be explained by the well-established fact that females have weaker neck muscles rendering them more susceptible to biomechanical injury [54]. At the same time, young females pay more attention to their health and promptly report their symptoms to coaches or medical personnel [55]. It is only fair to say that weakness of neck muscles is typical for children, and some specialists believe that sports training programs should include special exercise for neck strengthening that can protect against traumatic injury. However, we found no studies comparing the incidence and severity of SRC consequences between athletes and non-athletes that would factor in age.
CONCLUSION
Pediatric and adolescent sport-related brain concussions pose a challenge to contemporary science in general and sports medicine in particular. Recent research suggests that SRCs lead to profound changes in the body and require improved diagnostic approaches, as well as refined preventive and treatment strategies. The following problems remain unsolved to this day and demand special attention:
- new methods are needed for SRC diagnostics and the dynamic assessment of the brain’s functional state that would account for the age-related characteristics of young athletes; such methods could be based on neuroimaging techniques like functional magnetic resonance imaging, magnetic resonance spectroscopy, diffusion tensor imaging, functional magnetoencephalography, transcranial magnetic stimulation, etc.
- it is important to continue the search for and the development of special approaches to medication therapy and metabolic correction of typical delayed complications of SRC in young athletes, such as functional connectivity disorders, neurometabolic and circulatory changes, cognitive and sensor impairments;
- there is a need for training programs, protocols for SRC rehabilitation and medical surveillance to regulate return to active practice after injury;
- information leaflets for coaches should be prepared explaining what measures need to be taken if a young athlete sustains SRC and how SRC can be prevented.