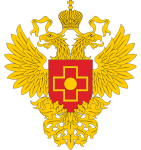
ORIGINAL RESEARCH
Neutralizing antibody creation technologies: case of SARS-CoV-2
1 Federal Scientific and Clinical Center of Specialized Types of Medical Care and Medical Technologies, Federal Medical Biological Agency of Russia, Moscow, Russia
2 Engelhardt Institute of Molecular Biology, Russian Academy of Sciences, Moscow, Russia
3 Research Institute of Pulmonology, Federal Medical Biological Agency of Russia, Moscow, Russia
Correspondence should be addressed: Vladimir P. Baklaushev
Orexovyj bul'var, 28, g. Moscow, 115682, Russia; ur.abmf-cknf@pv.vehsualkab
Funding: the study was supported financially by the Ministry of Science and Higher Education of the Russian Federation (contract #075-15-2021-1086, contract #RF----193021X0015, 15.IP.21.0015).
Since Köhler and Milstein developed the technology of production of monoclonal antibodies (hybridoma technology) in 1975 [1], hundreds of diagnostic and therapeutic antibodies have been designed, tested, registered, applied and discontinued [2]. This technology enabled production of MuromonabCD3, the first registered therapeutic antibody produced in mice [3]. Advancements of the recombinant DNA technology allowed humanization of mouse immunoglobulins, partial or complete, which was the next step in the development of the therapeutic (monoclonal) antibodies production technology [4]. Approximately simultaneously with the first human trials of mouse monoclonal antibodies the phage display technique was developed, first for peptides [5], then for antibodies [6]. Arguably, this technique became the most powerful tool enabling creation and "improvement of monoclonal antibodies; gradually, it replaced the hybridoma technology [2, 7]. Development of single cell sequencing yielded an alternative to phage display, a technique that allowed producing human monoclonal antibodies by cloning variable antibody fragments from a specific clone of plasma cells [8].
One of the most promising directions of medical application of human monoclonal antibodies is production of neutralizing antibodies (NAbs) and their use in prevention and treatment of socially significant infectious diseases. The COVID-19 pandemic made development of the SARS-CoV-2 neutralizing antibodies a particularly urgent task [9]. Over 20 NAbs have been designed, clinically tested and registered with the FDA and other regulating agencies since the beginning of the pandemic. Emergence of the new variants of SARS-CoV-2 rendered most NAbs ineffective, but a number of them have demonstrated a broad neutralizing activity against the most common subvariants of Omicron [8, 11]. Despite a significant decrease in the proportion of severe COVID-19 cases, NAbs still remain the most effective agents of etiotropic therapy, which is especially relevant for patients with oncological and hematological diseases and other primary and secondary immunodeficiencies [12].
The purpose of this review is to describe the current state of production of recombinant human antibodies using the example of neutralizing antibodies designed against SARS-CoV-2.
Antibody phage display
The antibody phage display method was developed independently by several groups of researchers, the first of which was the group of McCafferty from the University of Cambridge [6]. The method implies compilation of a phage library containing all possible variants of immunoglobulin variable regions. For this purpose, the antigen-binding antibody sequences are cloned into the pIII surface protein sequence of filamentous bacteriophages M13, fd or f1, which produces a number of unique clones, each of which presents a variable fragment of a certain specificity on its surface. The next step is to screen and select phages by this or that useful property, e.g., by the binding affinity to an antigen immobilized on the solid phase, followed by cloning of the selected sequences into vectors for antibody expression [6]. A phage library can be compiled from variable regions of immunoglobulin sequence of an immunized animal or human, but it can also be a random set of synthetic peptides [13].
Compared to other technologies, such as ribosome display [14], yeast display [15] or mammalian cell display [16], phage display libraries can have the variety of unique clones greater than 1011, with all of them stored for considerable periods in a state ready for screening with any antigen panel [7]. The variable fragments of antibodies in phage libraries can be antigenbinding Fab-fragments [17] or single-domain scFv-fragments (single chain fragment variable) [18, 19]. ScFv are monovalent fragments of antibodies with molecular weight of 25–27 kDa, consisting of the variable domains of heavy (VH) and light (VL) immunoglobulin chains connected by a peptide linker [20]. Fab are relatively large fragments of immunoglobulins that consist of VH, VL, CL, and CH1 domains. Compared to Fab, scFv offers a higher level of expression in phages, which is an advantage somewhat offset by the risk of loss of affinity upon conversion to Fab or full-length IgG [7]. There are variants of antibody phage libraries, those which include single-domain antibodies (human VH, camelid VHH, and shark VNAR, respectively); they are covered in a separate section below.
The antibody phage display enabled production of NAbs acting against HIV [21], anthrax toxin [22], tick-borne encephalitis [23], and, of course, SARS-CoV-2 [24]. The latter study demonstrated that phage display can produce high-affinity NAbs against the SARS-CoV-2 S protein with ID50 < 2 ng/mL from a semi-synthetic library of variable fragments of naive antibodies. Thus, compilation of an accurate CDR library of naïve B cells is the key factor ensuring stable pairing of VH and VL domains and, as a result, production of high-affinity neutralizing antibodies [24]. At the same time, it should be noted that far from all attempts at this task are successful, and the vast majority of highly active NAbs are obtained from samples of hyperimmune convalescents [8].
A noteworthy shortcoming of the canonical oligomeric antibody phage display technique is the fact that the resulting antibodies, as a rule, mismatch the natural repertoire, since they are generated from random pairs of VH and VL. One of the possible solutions to the VH/VL domain pairing problem involves use of phage libraries of the camelid family singledomain antibodies, the so-called nanobodies [25, 26].
Single-domain antibodies as a developmental platform for immunity preparations produced using the phage display technology
Single-domain antibodies, or nanobodies, are recombinant variable domains of VHH heavy chains derived from noncanonical immunoglobulins, with the Fab fragment consisting only of a shortened heavy chain, without a light chain. Normally, such antibodies are present in cartilaginous fish and the Camelidae in addition to the "classical" immunoglobulins G, which are comprised of two heavy and two light chains [25]. The key advantage of nanobodies is that the VHH domain, represented by a single polypeptide sequence, can be easily cloned in prokaryotic or yeast expression systems. The size of a nanobody is 12–15 kDa; they are highly soluble and capable of refolding after denaturating purification [25]. The increased solubility of VHH is the results of peculiarities of their amino acid composition. Compared to conventional antibodies, which have the VH and VL domains pairing interface dressed with hydrophobic amino acid residues, VHH have the hydrophobic amino acids in homologous regions replaced by more hydrophilic ones, which increases solubility of the recombinant products by reducing aggregation capacity [27].
With their small size and single-domain nature, nanobodies can penetrate structures inaccessible to full-length antibodies, and bind epitopes that are sterically shielded for conventional antibodies [25, 28–30]. Another reason behind the singledomain antibodies' capacity to penetrate steric shielding is the CDR3 loop in the VHH domain: it is longer than that of conventional antibodies, which allows single-domain antibodies to bind antigens located, for example, in the catalytic clefts of enzymes or in three-dimensional congruent regions of the ligand-receptor interaction [7]. With SARS-CoV-2 in particular, greater mobility allows single-domain antibodies to recognize the RBD of the S protein in its "down" conformation and disrupt the transition to the "up" conformation, rendering the protein nonfunctional [31] (see below).
The affinity of single domain antibodies is similar to that of conventional heavy and light chain antibodies, but nanobodies, unlike classical antibodies, are highly stable over a wide range of ionic strengths, pH values and temperatures [32]. The production of nanobodies in bacteria is cheaper than production of classical antibodies. The level of homology of framework regions of singledomain camelid VHH and human IgG3 subclass VH domains is high, which means the former can be easily humanized and retain their functional properties in the process [25]. All of the above translates into the prerequisites justifying research and practical application of recombinant single-domain antibodies both for diagnostic and therapeutic purposes [33, 34].
Bi- and trispecific/valence nanobodies
Small size of single-domain antibodies awards them rapid kinetics in the systemic circulation; they are eliminated through the kidneys within a few hours. On the one hand, this is an advantage usable, for example, in development of radioisotope diagnostic tools [26]. On the other hand, it limits the use of nanobodies as preventive and therapeutic agents and necessitates additional efforts aimed at increasing their half-life in the bloodstream. The solution to the problem of rapid elimination of nanobodies from the body is their oligomerization and/or creation of bi- and trispecific antibodies. Heterodimerized and bi- and trivalent nanobodies have significantly longer pharmacokinetic persistence. An example thereof is the ALX-0061 heterodimeric bispecific nanobody produced by Ablynx; it consists of a high affinity VHH domain binding the IL6 receptor with an affinity coefficient of 0.19 pM and a VHH domain specific to serum albumin. The latter brings the half-life of the heterodimeric complex up to 6.6 days, with the molecular weight of the former of 26 kDa [35] and this is clearly not the ultimate limit. This high an affinity of the ALX-0061 nanobody is the result of "affinity maturation", also enabled by phage display; the technology increased the affinity 200-fold compared to the initial VHH domain [35]. Another example of a therapeutic antibody produced through heterooligomerization of nanobodies is Ozoralizumab, a humanized bispecific trivalent antibody including two TNFα-binding VHH domains and one serum albumin-binding VHH domain [36]. Supplementing the bi- and trivalent antibodies with VHH domain that binds serum albumin can be considered one of the standard approaches to augmentation of half-life of recombinant nanobodies [37].
Oligomerization of VHH domains to create bi- and trivalent antibodies not only increases the half-life of these antibodies but also enhances their functionality by building up the avidity of such antibodies [38]. Another way to boost half-life and functionality of nanobodies is through creation of fusion proteins with the Fc fragment of human immunoglobulins. A nanobody modified with an Fc fragment has a significantly longer halflife in the bloodstream; the fusion also promotes activation of the Fc-mediated effector functions (antibody-dependent cellmediated cytotoxicity, complement-dependent cytotoxicity, etc.) [39, 40].
Virus neutralizing nanobodies
In the pre-pandemic era, different groups of researchers designed nanobodies neutralizing the respiratory syncytial virus [41], MERS-CoV [42], pandemic variants of the influenza (H1N1 [43], H5N1 [44]), as well as multidomain broad-spectrum influenza neutralizing nanobodies that bind hemagglutinin [45]. When the SARS-CoV-2 pandemic began, this technological knowledge enabled development of neutralizing nanobodies acting against the new pathogen. For example, yeast display technology [46] allowed producing synthetic neutralizing mNb6-tri nanobodies targeting the SARS-CoV-2 S-protein. These nanobodies were shown to bind with the S trimer in the "down" conformation, stabilize it in this inactive form and thus make interaction with the ACE2 impossible [31]. Genetic engineering optimization gave trivalent mNb6-tri antibodies femtomolar affinity and picomolar concentration for complete SARS-CoV-2 virus neutralization. These antibodies retain their properties having undergone lyophilization, heating, aerosolization, and thus can be used in inhalations for the purpose of virus neutralization in the bronchoalveolar tree [31].
A panel of RBD-specific nanobodies was obtained from a library of VHH phage displays created from B-cells of a Bactrian camel immunized with recombinant RBD [47]. Three clones, P2C5, P5F8, and P2G1, were selected with in vitro virus neutralization test as completely suppressing the cytopathic effect of SARS-CoV-2 at concentrations of 12–48 nM. Seeking to further improve antivirus properties of the antibodies, a group of researchers produced homodimeric and heterodimeric forms of nanobody clones that had 100-fold (minimum, some were more potent) higher virus neutralizing potency compared to monomers [47].
The new variants of SARS-CoV-2 that are better at avoiding virus-neutralizing antibodies add urgency to the task of creation of broadly neutralizing antibodies that bind all possible SARSCoV-2 variants. At least one option thereof has been produced with the help of the single-domain antibody technology. A group of researchers immunized a llama alternately with the S protein of SARS-CoV-1 and MERS-CoV, then derived a phage library of antibody variable domains and screened it against the S protein of SARS-CoV-2, among other things. They found the VHH72 nanobody, which boasts high cross-neutralizing activity against SARS-CoV-1 and SARS-CoV-2. The researchers have created a bivalent antibody based on VHH72 as a fusion protein with the Fc fragment of human Ig, and shown its promise as a possible base for a broadly neutralizing antiviral drug [48]. Phage display and VHH have enabled design of other virusneutralizing nanobodies that inactivate SARS-CoV-2 [49].
Thus, using the single-domain antibody technology, a number of promising homo- and heterodimeric NAbs were produced, all of them showing promise as base for an etiotropic drug for treatment and prevention of COVID-19.
Production of recombinant human antibodies from individual B cells
From the historical and methodological points of view, the approach to production of human monoclonal antibodies most advanced currently is direct isolation of specific B cells followed by sequencing of the genomes of individual cells and identification of variable fragments of MAbs produced by them [50]. There are three variations of this approach, each with its own methodology of the first stage (identification and cultivation of the antigen-specific clone of B cells). For example, the hybridoma technology allows producing hybridomas of target B cells with myeloma cells and carrying out selection on the HAT medium, and then collect hybridomas with the desired specificity (1); or, isolate, culture and collect memory B cells (2); or, directly isolate memory B cells with a target BCR interacting with a fluorescently or magnetically labeled antigen, and then analyze the repertoire of specific B cell clones using the singlecell sequence technology (3). The latter option is the most advanced one; it allows producing panels of specific NAbs in a relatively short time [8]. Antigen-specific memory B cells can be obtained from the plasma of hyperimmune patients or from transgenic mice carrying human immunoglobulin loci and producing fully human antibodies in response to immunization with the target antigen [51]. There is a number of technological solutions that improve performance of screening of individual antibody-producing cells, like microfluidic sorting of B-cells with assessment of BCR specificity, followed by bar-coding of VH and VL pairs and high-throughput sequencing [52].
The advantage of the new technology is that its result does not depend on the diversity of the library of variable domains, but, at the same time, it is always a variant of the natural repertoire of antibodies, which means an acceptable safety profile and a significantly lower probability of non-specific (offtarget) interactions with its own antigenic determinants [50]. Along with single-cell NGS sequencing, high-performance technological solutions enable simultaneous analysis of hundreds of different clones of memory B-cells secreting antibodies of a given specificity and subsequent selection by various useful properties (affinity, avidity, overlapping antigenic epitopes, etc.) [8, 52].
The approach implying production of NAbs from individual clones of B cells the with the help of the single cell sequence technique has proven to be highly efficient in creation of broadly neutralizing antibodies that block the CD4 binding site in the V1/V2 and V3 regions of gp120, as well as HIV gp41 [53, 54]. In addition, this technology is behind design of MAbs against cytomegalovirus [55], S-antigen of hepatitis B virus (HBsAg) [56] and a large number of NAbs against the SARS-CoV-2 S protein [57–60].
Some of the first neutralizing antibodies REGN10933 (casirivimab) and REGN10897 (imdevimab) were obtained by applying the single B cell assay technology to the material collected from immunized humanized mice and convalescents after COVID-19 [58]. NGS sequencing and 3D mapping of antigenic epitopes (done with hydrogen-deuterium exchange mass spectrometry) enabled analysis of a panel of more than 200 virus-neutralizing antibodies, which ultimately yielded four antibodies characterized by non-overlapping epitopes. Used in a cocktail, the pairs of these antibodies effectively neutralized all SARS-CoV-2 variants known at that time.
A similar study aimed at creation of a panel of SARS-CoV-2 virus-neutralizing antibodies was conducted in 2021 [8]. As a result of NGS sequencing of clones of B cells from patients that had severe COVID-19, 18 high-affinity antibodies to RBD with KD in the range of 0.47–13.3 nM and virus-neutralizing capacity were produced; four of them have shown 100% virus neutralization at concentrations below 16 ng/ml [8]. The next step was to do a competitive analysis of interaction of the obtained antibodies with a panel of commercially available neutralizing antibodies with a known 3D structure of the antigenic epitope. COVA2-15 [59] and COV2-2504 [60] can be named here in addition to the already mentioned REGN10933 (casirivimab) and REGN10897 (imdevimab) [58]. The results of the competitive analysis and a series of SARS-CoV-2 RBD (with known point mutations) viral neutralization experiments allowed an accurate identification of antigenic epitopes of the obtained ultra-neutralizing antibodies. By combining antibodies complementing each other NAb cocktails that effectively neutralize all studied variants of SARS-CoV-2 were mixed. This experience shows that a comprehensive panel of broadly neutralizing antibodies from individual memory B cells that covers all possible antigenic epitopes and avoids point mutations allows compilation of effective virus-neutralizing cocktails against any new variant of SARS-CoV-2. If necessary, the panel of broadly neutralizing antibodies can be supplemented with targeted mutagenesis of antigen-binding sites.
There was developed a number of microfluidic platforms that significantly increase cloning throughput and single antibody expression. One of them is the 10x Genomics platform: drops containing one antibody-producing cell each, as well as a lysis buffer with microbeads coated with bar-coded primers, are generated in a microfluidic device to encode cDNA of specific native pairs of VH and VL domains [61].
A recent advancement is LIBRA-seq (linking B cell receptor to fntigen specificity through sequencing) a technology enabling high-throughput BCR screening by binding B lymphocytes to antigens barcoded using oligonucleotides, followed by NGS sequencing [62]. Using this technology, several thousand B cells from HIV-infected patients were screened for antigenic specificity, which yielded confirmation of the predicted specificity for antibodies to HIV, influenza and SARS-CoV-2, including known and unknown NAbs.
The MAbs produced using the single B cell technology can be genetically modified in the same way as antibodies obtained by phage display. For example, it is possible to modify the Fc fragment to increase the circulation of antibodies in the bloodstream. Among the most advanced modified antibodies to SARS-CoV-2 is sotrovimab, also known as VIR-7831 and GSK4182136, designed by Vir Biotechnology and GlaxoSmithKline, approved by the FDA in 2021. The Fc fragment of sotrovimab includes amino acid substitutions M428L and N434S (LS modification), which prolongs its half-life [63]. Another example is AZD7442, a cocktail of tixagevimab
(AZD8895) and Cilgavimab (AZD1061) [64] compiled by AstraZeneca. Both NAbs, combined, have engineered Fc domains including L234F/L235/P331S substitutions (TM modification), resulting in little or no binding to various FcyRs or C1q complement protein, and insignificant to non-existent manifestations of the effector function in vitro [64].
Prospects of artificial intelligence (AI) in further development of the human antibody design technologies
Today, there is an already established candidate for the role of a fundamentally new human antibody modeling technology: AI-enabled in silico antibody structure prediction [65]. The AlphaFold2 neural network launched in 2021 can predict spatial structure of proteins from their primary sequence with accuracy at the atomic level [66]. AlphaFold2 is the first successful application of machine learning to the task of modeling tertiary structure of a protein. AlphaFold2 relies on the so-called multiple sequence alignment (MSA), analyzing information about the pairing of amino acid residues and structural templates for the primary sequence [67].
The AbAdapt service was developed specifically to predict the 3D structure of antibodies and antigenic epitopes; it combines structural modeling of antibodies and antigens with modeling of their interaction. By default, AbAdapt takes primary sequences as input and uses the Repertoire Builder [68], a high performance antibody structure modeling service. In 2022, AlphaFold and AbAdapt were merged to build the AbAdaptAF system [69], which more accurately predicts the structure of paratopes and antigenic epitopes specific to antibodies. The authors used the service to analyze the virus-neutralizing antibody to RBD domain of SARS-CoV-2 and showed that their system is best in modeling the antigen-antibody interaction. The recently built Ablooper [70] and DeepAb [71] specialized neural networks have proven to have a better throughput than the Rosetta Antibody Benchmark and AlphaFold2 networks.
In August 2022, there was launched the NanoNet neural network, which is optimized to predict the 3D structure of VHH [72]. Its architecture includes a high-precision neural network (CNN) and two additional neural networks (ResNet). The first, ResNet, analyzes scaffolds and hypervariable CDR cycles, while the second perceives interactions between amino acid residues. Comparison of NanoNet and AlphaFold2 in terms of prediction of 3D structure of the known 16 VHHs deposited in the PDB in 2021, which means they were not part of the dataset AlphaFold2 was trained on, revealed that NanoNet offered better accuracy on the atomic level. Thus, NanoNet is a very promising new tool for modeling the structure of VHH; it is used, inter alia, to optimize the predictions of structure of CDR3 loops that neutralize VHH acting against SARS-CoV-2 [73].
It can be concluded that today, it is already theoretically possible to create high-affinity variable domains of antibodies in silico, i.e., without actual use of B cells and immunization. There is no doubt that in the future, selection of a high affinity sequence for specific antigenic epitopes with the help of machine learning will be a routine method of production of human antibodies.
CONCLUSION
Currently, the monoclonal human neutralizing antibody production technologies rely on phage display and derivation of antibodies from individual B cells. Each technology has its own advantages and limitations. Phage display allows rapid screening of phage libraries with antigen-binding site sequences, such a screening done against novel antigens. The VHH single-domain antibody technology allows creating bi- and trispecific antibodies, optimization of the affinity and capacity to neutralize new variants of SARS-CoV-2. The technology for obtaining antibodies from individual B cells, enhanced by high-throughput screening based on microfluidics and NGS sequencing, enables compilation of panels of virus-neutralizing antibodies that can be combined to cover any SARS-CoV-2 RBD modification. Future advancements of the monoclonal antibody production technology involve neural networks and machine learning that are used to predict the primary structure of variable domains of antibodies based on the tertiary structure of the target antigen.