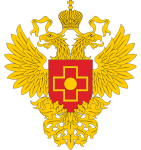
This article is an open access article distributed under the terms and conditions of the Creative Commons Attribution license (CC BY).
REVIEW
Structural and functional changes in the brain of cosmonauts under the influence of microgravity
1 Federal Research and Clinical Center of Space Medicine of Federal Medical Biological Agency, Moscow, Russia
2 Lomonosov Moscow State University, Moscow, Russia
Correspondence should be addressed: Konstantin V. Latartsev
Shchukinskaya, 5, st. 2, Moscow, 123182, Russia; moc.liamg@vestratal.k
Funding: the review was supported financially under the State Task “Structural and functional changes in the human brain and their effect on operator actions at different timepoints of the term of adaptation to simulated microgravity” (code “Cerebrum A”).
Author contribution: Latartsev KV — analysis of source materials, article authoring and editing; Demina PN — search for source materials, analysis thereof; Yashina VA — search for source materials, analysis thereof; Kaspransky RR — study conceptualization, search for source materials, manuscript editing.
During a spaceflight, a number of factors specific thereto cause structural and functional rearrangements in the brain, same as in other organs and tissues of the body [1]. Presently, such attributes of a space mission as microgravity, prolonged isolation, radiation, disruption of sleep patterns and circadian rhythms are known to affect human psychophysiology by disturbing operation of various parts of the brain [2–4]. In microgravity, gravitational pull of the nearest space object is extremely small, and physical items seem weightless; the associated stable background acceleration is of the order of 1 × 10–6 g. To date, there has been accumulated a large amount of information yielded by the studies designed to investigate the effect of microgravity on physiology of the brain. The respective papers describe both structural and functional changes occurring in the brain under the influence of this spaceflight factor: disorders of the vestibular function, neuroplastic adaptation, redistribution of fluid in the body (shifts to the upper part of the body, subsequently elevates intracranial pressure (ICP) and triggers many concomitant effects). Changes in the functioning of the brain that result from adaptation to the conditions of spaceflight can become a problem during deep space missions, therefore, studying the effect of microgravity as a phenomenon integral to spaceflights, is an important task.
The effect of microgravity on the volumetric characteristics of brain structures
Redistribution of fluid in the body is one of the main problems associated with a spaceflight. Earth gravity gradients hydrostatic pressure in a human being: its value gradually increases from the head to the lower extremities. In microgravity, there is no force to effect this gradient, and weaker forces (surface tension, etc.) gain control over the behavior of fluid in the body, eventually redistributing it. Apparently, the upper body's vascular network, spinal membranes, interstitial tissues, and possibly the lymphatic system receive and store most of the redistributed fluid [5]. This entails various changes, including those in the central nervous system, like the previously registered narrowing of the cerebrospinal spaces around central and calcarine sulci and upward displacement of the brain inside the skull [6]. At the same time, post-flight examinations and observations revealed expansion of the cerebrospinal fluid spaces along ventral surface of brain and ventricles, gray matter contraction in the orbitofrontal and temporal cortices with the total volume of gray and white matter remaining unchanged [7, 8], and growth of the volume of free water in the frontal, temporal and occipital lobes [9]. Moreover, it was found that long-term spaceflight (LTSF) boosts the dynamics of cerebrospinal fluid (CSF) in cerebral aqueduct, causes its volume to grow and brain to expand. Previous research revealed LTSF to be associated with a progressive increase in mean and pulse ICP, conditioned by the mentioned greater volumes of the CSF and brain matter, and reported white matter expansion and pituitary deformity in 6 out of 11 crew members after a long-term space mission [10]. One study sought to assess volume and morphology of periventricular white matter (PWM), and found that it increased in first-time cosmonauts, whereas no such phenomenon was registered in their experienced counterparts [11]. Other studies, which established a link between spaceflight duration and the degree of enlargement of cerebral cavities, confirm these findings [12].
However, fluid redistribution is not the only reason for changes in the volumetric characteristics of the brain. There are also microstructural changes, which will be discussed in the following sections.
The effect of microgravity on the sensorimotor system
Prolonged stay in microgravity impairs human cognitive abilities, causes asthenia, and disrupts spatial orientation. In addition, as shown by post-flight and simulation studies, LTSF adversely affects performance and learning ability, both dependent on the higher mental functions [13, 14]. It is clear that an abrupt change in gravitation can have negative consequences for a human being's sensorimotor control, since it developed in the conditions of Earth. Researchers have set up experiments to investigate the effect of hypergravity (simulated) and microgravity (simulated and real), and learned that both have a negative impact on the accuracy and speed of hand movements, with more noticeable changes registered for upward arm movements than for downward movements. Shoulder girdle's muscles have been shown to work differently, too, the respective alteration conditioned by the degree of parallelism between the gravity vector and the direction of application of force exerted by these muscles. For example, pectoral muscle worked perpendicularly to gravity, and in the experiment, gravitational changes affected it the least [15, 16]. These findings found confirmation in subsequent studies. Presumably, altered gravitation could have forced the sensorimotor system to perceive weight changes as an increase or decrease of the mass of the held object, as well as that of the hand itself, when these parameters have in fact remained unchanged. Microgravity made hand movements slower and lasting longer, hypergravity — less accurate and lasting longer; the latter effect is probably conditioned by a more concentrated conscious cognitive control effort. The increased feedback seems to have helped compensate for the decreased accuracy [17]. These experiments show that in microgravity, there is a need for additional cognitive control of movements, which, apparently, gives rise to a deficit of neural resources due to sensorimotor adaptation to microgravity. This process, in turn, degrades the ability to simultaneously perform cognitive and motor tasks when in space [18]. Terrestrial experiments simulating individual effects weightlessness also show rearrangements in sensorimotor and spatial working memory that hinder the ability to solve complex tasks and cause psychoemotional shifts [19, 20].
The effect of microgravity on structural changes in the brain
Adaption to the peculiarities of movements and performing various tasks in microgravity is accompanied by structural changes in the brain departments and pathways that are mainly associated with motor activity. Functional MRI has revealed that prolonged exposure to weightlessness promotes rearrangements in the vestibular and motor regions of the brain, with alterations registered predominantly in the cerebellum, cortical sensorimotor and somatosensory regions, as well as vestibular pathways, accompanied by the changes in the number of synapses and axonal degeneration [21, 22]. It was also found that microgravity causes microstructural changes in the brain in general and sensorimotor tracts in particular [23]. In 2016, scientists noted a decrease in the amount of gray matter in the frontal and temporal lobes, in the orbitofrontal cortex, as well as in the bilateral medial sections of the middle cerebellar peduncles, and changes in some regions of the brain were significantly more pronounced after LTSF [24]. As for white matter, changes therein were detected in the right superior and inferior longitudinal fascicles, corticospinal tract and cerebellar peduncles [9]. In 2022, significant microstructural changes were identified in the corpus callosum, arcual fascicle, corticospinal, corticostriar and cerebellar tracts [23]. These discoveries point to adaptive neuroplasticity mechanisms that are activated when a person's environmental conditions alter. A greater volume of white matter in the cerebellum registered after spaceflight also clearly confirms activation of these mechanisms [25]. Russian scientists have also demonstrated adaptive neuroplasticity in Russian cosmonauts [26].
At the same time, brain rearrangements occurring in the context of an LTSF reduce the individual's adaptability to the conditions of Earth gravity. Post-flight examinations of cosmonauts have revealed hindered operation of the precentral and postcentral gyri, responsible for voluntary movements and proprioception, respectively, and maloperation of the cerebellum, which governs movement coordination. Damage to these areas of the brain leads to somatosensory disorders, problems with the accuracy and speed of voluntary movements, and obstructs movement synchronization [27]. Observations of cosmonauts show that on the day of landing they experience ataxia, manifested in impaired coordination of movements and pose maintenance, which indicates dysfunction of the vestibular system and impaired proprioception. Impaired coordination of movements is recorded three days after the flight, too, but without dizziness [2]. The post-spaceflight changes in human sensorimotor characteristics, including degraded motor activity, balance and fine motor skills, are well-documented [18]. Investigation of the adverse effects of microgravity associated with alterations in somatosensory and vestibular signals revealed significant changes in the volume of gray matter. Antiorthostatic hypokinesia triggered growth of its volume in the postparietal and shrinkage thereof in the frontal regions of the brain. These structures govern control and coordination of voluntary movements, sensorimotor coordination, and processing of sensory information. In addition, functional mobility and the ability to maintain balance while standing have been observed to deteriorate. Such changes are associated with a greater amount of gray matter in the region that includes quader, precentral and postcentral gyri, which are responsible for sensory perception, motor control, and orientation in space and time [28].
The effect of microgravity on functional changes in the brain
The listed adaptive mechanisms condition functional connectivity of the brain regions after exposure to microgravity. For example, structural connectivity of white matter changes in areas involved in visual-spatial data processing, vestibular function, and motion control, suggesting that the processes requiring prefrontal multimodal integration of sensory signals may be at risk during spaceflight [9]. Immediately after the LTSF and 8 months later, there has been registered a persisting deterioration of connectivity in the posterior cingulate cortex and thalamus, with the respective indicator increasing in the right angular gyrus. Connectivity in the bilateral insular cortex decreased after spaceflight, but returned to normal by the time of the next examination. The study shows that the altered gravitational environment affects functional connectivity longitudinally in multimodal brain centers, reflecting adaptation to unfamiliar and contradictory sensory information peculiar to microgravity [26]. Another study detected deteriorating connectivity in the right insular lobe associated with afferents of otolith organs and semicircular canals, as well as between the cerebellum's left hemisphere and the motor cortex's right zone. The conclusions that the motor cortex is less connected during rest and gets more activated in the context of an active task can be considered a compensatory adaptive response to microgravity, as well as a mechanism of adaptation triggered in the early post-landing period [27]. In one study, scientists sought to determine correlations between changes in the brain and changes in the performance of spatial working memory (SWM) before and after flight; they did not register any significant impact of LTSF on the performance of SWM or brain activity, but observed significant changes in brain connections. Thus, superior occipital gyrus was found to communicate with the rest of the brain less effectively. Testing of SWM also revealed poorer connection between left middle occipital gyrus and left parahippocampal gyrus, as well as left cerebellum and left lateral occipital cortex [29]. In addition, another study recorded intra- and interhemispheric anti-correlations between temporoparietal junction and supramarginal gyri, which indicate a change in both vestibular functions and functions associated with consciousness [30]. Prolonged stay in antiorthostatic hypokinesia led to a deterioration of balance and motor activity, as well as significant changes in the functional connectivity of the motor, somatosensory and vestibular regions of the brain [31]. Moreover, some of these changes were largely associated with rearrangements in the sensorimotor and spatial working memory, which suggests that neuroplasticity mechanisms may contribute to adaptation to simulated microgravity [20]. For cosmonauts, one of the most important problems associated with impaired sensorimotor and cognitive functions is space motion sickness, which occurs when a person undergoes acute adaptation to microgravity. Examinations of the brain during nausea caused by two different types of stimuli have revealed dipoles in the area of cerebral cortex (lower part of the frontal gyrus) measuring 2–3 cm in diameter, which signals of activation of neurons. Severe nausea meant more dipoles than milder nausea. Thus, nausea caused by the vestibular system affects the prefrontal areas of the brain that are associated with autonomic regulation of emotions, which implies that motion sickness can have an effect on those prefrontal areas and disrupt autonomic mechanisms [32]. Clearly, transformation of the pathways participating in cognition and processing of sensory information can negatively affect the ability to pilot and dock a spacecraft or perform other operations that also require spatial memory. In 2020, there have been designed studies to determine the effect of microgravity on higher cognitive functions. However, they yielded contradictory results: some reported deterioration thereof, others — improvement, yet others — lack of changes [33]. A year earlier, there was conducted a study that observed twin astronauts before, during, and after a one-year mission to the International Space Station (ISS). This study has shown that cognitive tasks proved to be significantly more difficult to solve only after the flight, but the earlier periods of the experiment the ability of the participants to tackle them remained unchanged [34].
Causes and mechanisms of microstructural changes in the brain
Based on the analysis of the literature, it can be concluded that adaptive neuroplasticity and fluid redistribution are considered to be the two main microgravity-associated factors that drive microstructural changes in the brain. Presumably, neuroplastic adaptations mainly occur in the systems that govern motor activity. Based on the already existing theory of how cerebellum processes information, it can be assumed why microgravity triggers certain changes.
The cerebellum of a mammalian combines information from a variety of sensory systems with motor commands and sends projections to the motor and premotor regions of the cerebral cortex, as well as to the nuclei of the brain stem and spinal cord. Many areas of the cerebellum receive inputs directly from vestibular afferents and vestibular nuclei. Presumably, cerebellum generates an internal model that predicts somatosensory consequences of the movements performed, after which the predicted and actual consequences of motor behavior are compared. Currently, it is believed that the cerebellum calculates differences and generates an error signal that controls recalibration of motor centers [35]. On Earth, the body is constantly exposed to gravity, which leads to continuous stimulation of otolith organs and proprioceptors. Studies show that some of the Purkinje cells in the cerebellum encode head positions and their changes relative to the direction of gravity. Apparently, orientation in space factors in constant gravity, so researchers believe that the internal model of motion calculated inside the cerebellum also accounts for this force [35]. However, spaceflights imply microgravity, which translates into a discrepancy between sensory consequences of movements modeled by the brain and the real sensations. Consequently, this triggers rearrangements in the vestibular signal processing system that enable adaptation to the new environmental conditions. In this connection, a study reported an increase in the mass of otoliths and the number of hair cells in the first weeks of spaceflight, a short-term increase in the sensitivity of the vestibular pathways (returned to normal over time), and changes in the morphology of the Purkinje cell dendrites and synaptic organization of afferent mossy fibers [36].
From a molecular point of view, microstructural rearrangements occur under the influence of various signaling protein molecules. One of the key protein factors that play a part in the mechanisms of neuroplasticity is the brain0derived neurotrophic factor (BDNF), different isoforms of which positively and negatively contribute to the maintenance of brain homeostasis. For example, pro-BDNF stimulates apoptosis and negatively affects remodeling of neurons, eliminating an excessive number of maturing or damaged cells and ineffective neural connections, while m-BDNF (m — mature) supports neuro- and gliogenesis, branching of dendrites and development of the dendritic processes. Multidirectional influence of these isoforms allows precise control of dynamic balance, which is necessary to maintain brain homeostasis [37]. In an animal experiment (rats), it was shown that learning boosts expression level of BDNF isoforms, as well as tyrosine kinase receptor B (TrkB), which mainly acts as a BDNF receptor [38].
Regular physical exercises by cosmonauts should also be taken into account. Some data indicate that physical exertion has a positive effect on neuroplasticity, increasing expression of certain proteins; in particular, high-intensity short workouts boost production of BDNF, TrkB, and pCREB [39]. Phosphorylated transcription factor CREB activates transcription of the BDNF gene and acts as a marker of LIM kinase 1 (LIMK1) activity, which blocks actin depolymerization, with subsequent restructuring of dendrite spikes and supported neural plasticity [40]. Other studies have shown that regular exercising enhances neuroplasticity by increasing the expression of insulin-like growth factor 1 (IGF 1), which is important for the development and maintenance of brain functions, as well as BDNF protein and the positively correlated vascular endothelial growth factor (VEGF), which mediates angiogenesis in the brain. Moreover, it was found that smaller BDNF expression has a similar effect on the VEGF, with ultimate slowdown of angiogenesis [41].
The described changes lead to enhanced neuro-, angio- and synaptogenesis and, apparently, cause structural and functional rearrangements in the brain, including growth of the volume of gray matter in the cerebellum, hippocampus, basal ganglia and some parts of the cortex, and changes in the volume of white matter [42].
In addition to molecular effects, it is necessary to note the general physiological effects mediating microstructural changes in the brain. For example, a simulated microgravity experiment (dry immersion) has shown a slowdown of blood flow in 32 cortical and subcortical regions after 5 days [43]. Presumably, this effect may be the result of the narrowing of cerebral arteries caused by displacement of brain fluid: in the early days of a spaceflight, cerebral blood flow increases along with intracranial pressure, which leads to chronic vasoconstriction of the arteries and subsequent hypertrophy. Prolonged vasoconstriction, combined with histological changes, is probably responsible for stronger vascular resistance and weaker total blood flow in the brain [43]. Moreover, there is evidence of plasma volume decreasing in microgravity, which also contributes to a decrease of cerebral blood flow [44]. In addition to the general blood flow decrease, there is a redistribution of blood supply in various parts of the brain. Inter alia, regional blood flow in the basal ganglia was registered decreasing, a phenomenon explained by intensification of operation of these areas due to their central role in information processing; however, there are assumptions of a potential modification of neurotransmitter metabolism, which is largely unexplored in the context of microgravity [43]. Such changes in the nature of blood supply are presumably closely related to the structural changes in the brain. It has been shown that transformations in the primary sensorimotor cortex, basal ganglia and cerebellum do not entail changes in the proportion of free fluid, which shows the importance of further research efforts designed to fully clarify the exact nature of the effects of spaceflight on brain structures [21]. There are other factors that affect the brain of the cosmonaut, and they need to be taken into account, too. Thus, it was shown that one of the conditions of spaceflight — prolonged isolation — decreases the volume of the hippocampus and brings down the correlated concentration of BDNF [45]. Moreover, a decrease in the volume of the dentate gyrus of the hippocampus was also associated with deteriorating cognitive performance as shown by spatial processing and selective attention tests [45]. In addition, it is known that ISS crew members often complain of headaches, visual and cognitive impairments that correlate with CO2 levels, and, judging by the existing studies, the redistribution of fluids in microgravity in combination with an increased CO2 content in the atmosphere affects nervous functions and cognitive performance [46]. In this regard, it should be mentioned that cosmonauts on the ISS have increased expression of hypoxiainduced factor alpha-1 (HIF-1a), which, in turn, pushes up the concentration of VEGF-1, a protein the expression of which correlates with BDNF [47]. All these phenomena indicate wide network interactions of molecular genetic effects resulting from the complex of various factors of spaceflight that are to be investigated.
CONCLUSION
Thus, we can assume that the functional and structural transformations observed in cosmonauts' brains are consequences of molecular, genetic, and other intracellular changes, which, in turn, are caused by living conditions altered by spaceflight. The most influential factor is microgravity, which causes the redistribution of fluid in the body and neuroplastic adaptation of sensorimotor systems to changed conditions. There are, however, other factors of spaceflight that also affect cosmonauts. For example, prolonged isolation translates into weaker expression of BDNF, and hypoxia occurring in stagnant zones of the ISS, where the level of CO2 is increased, leads to greater concentrations of VEGF-1, which is associated with BDNF. Clearly, other conditions cause their own molecular effects, which are interconnected. There is a complex interaction following the pattern of an extensive network, where each external factor adds its contribution. Determining the specific roles of each of the factors of spaceflight is an extremely labor-intensive task that is yet to be tackled. Solving this task would allow a more accurate and detailed study of the influence of spaceflight factors on the human central nervous system, which is necessary, first of all, to develop preventive measures aimed at minimizing the negative consequences of such flight. Investigation of these issues is of special interest, since it is the improvement of health and efficiency of crew members that largely determines the success and safety of future mission, including flights to deep space, Mars, and asteroids, which are the immediate goals defined in the strategy and concept of the development of the Russian space industry.