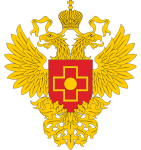
This article is an open access article distributed under the terms and conditions of the Creative Commons Attribution license (CC BY).
REVIEW
Multipotent mesenchymal stem cells: prospects for use in the treatment of injuries sustained in the Far North
National Medical Research Center for Rehabilitation and Balneology, Moscow, Russia
Correspondence should be addressed: Marina Viktorovna Volkova
Novy Arbat, 32, Moscow, 121099, Russia; ur.tsil@avoklov.hcetoib
Acknowledgements: the authors express their gratitude to Jan Borisovich Kovalevsky, General Director of Orion Chemical Company, for his assistance in writing the article.
Author contribution: Volkova MV — scientific justification, data analysis, manuscript authoring; Eremin PS — manuscript editing; Markov PA — project supervision, manuscript editing.
Skin is a vital organ that prevents penetration of foreign agents into the body and participates in metabolism. In the absence of surgical treatment, deep and extensive skin lesions that accompany serious wounds (burns, frostbite, etc.) heal unevenly in most cases, with subsequent fibrosis, scars, and wound becoming chronic, as well as increased risk of amputation, sepsis, and death of the patient [1].
Bones are an equally complex organ that has protective, shaping, and mechanical support functions in the body as a whole. [2]. Bone tissue is a highly dynamic structure that constantly undergoes remodeling needed to adapt to changing mechanical loads and repair the developing fatigue fractures. Despite the high regenerative capacity thereof, bone loss and its subsequent restoration are important problems in orthopedics and related fields: their clinical and economic impacts are significant, because patients take long time to recover and risk losing ability to work [3]. Bone is a unique tissue that regenerates constantly and is capable of complete regeneration, with healed fractures being the vivid evidence. However, in some cases, damaged tissues require greater regenerative potential than what is normally available in the body. As a rule, such cases involve defects of critical magnitude brought by orthopedic or maxillofacial surgery, injuries, infections, and tumor resections; every such situation requires clinical intervention and endogenous stimulation of bone regeneration [4].
Every year, more than 10 million injuries — from skin damage through bone fractures to damage to internal organs — are registered in the Russian Federation. About 4 million more people have diseases of the skin and subcutaneous tissue, about 5 million suffer from conditions affecting musculoskeletal system and connective tissues [5]. A significant proportion of injuries sustained in the context of domestic, road traffic, and industrial incidents involve skin, muscle, and bone damage. In wartime, these figures increase manyfold and incorporate gunshot, shrapnel, and mine blast wounds.
Currently, the Arctic region, which has global geopolitical significance, is being developed with transport corridors, hydrocarbon production facilities, etc. [6]. These activities in the Far North increase the risks of injury and disability, as the Arctic affects all systems of the body significantly. Difficult working conditions, high risk of injury, and remoteness from inpatient medical institutions underpin the urgency of development of the new methods and materials that stimulate regeneration of hard and soft tissues, including in cases of combined genesis [7].
Preparing this review, we searched relevant papers in Google Scholar and PubMed. The key words cited above were used in the search for publications.
The review aims to compare promising approaches to treatment of skin and bone tissue injuries sustained under extreme conditions that employ mesenchymal stem cells.
The specifics of inflammatory and regenerative processes in the extreme climatic conditions of the Arctic
Climatic and geographical factors of the Arctic have a significant effect on human body, leading to a complex restructuring of the homeostatic systems and activation of all physiological reserves [8]. The so-called polar stress syndrome, which is state of specific physical strain, affects people who have recently arrived in the region and undergo adaptation to the extreme conditions [9].
Low temperatures are one of the key climate-driven factors peculiar to the Far North and the Arctic. They activate mechanisms boosting production of heat and preventing its loss [10]. Normal internal body temperature is 35.6–37.8 °C. When it drops below 35 °C, the person suffers hypothermia. Prolonged or deep hypothermia can translate into irreversible damage, up to loss of motor function and death [11–12].
Hypothermia is not the only dangerous condition risked in the Far North and the Arctic. Extreme weather and climate of the region strain respiratory system excessively and increase the risk of pulmonary pathologies [13]. Cold air causes activation of a number of protective physiological mechanisms, including respiratory rate slowdown and reflexive shallow breathing, with the functional residual lung capacity growing in the background. Upper respiratory tract actively loses moisture and heat necessary to dampen and warm the inhaled air [14]. Changes in the respiratory organs and tissues negatively affect the entire body, with efficiency of oxygen transport and utilization dropping in the first place, which triggers oxidative stress and increased formation of free radicals, including reactive oxygen species (ROS) [15–17]. Excessive accumulation of ROS leads to mitochondrial dysfunction, further exacerbating the effects of the said stress, mediating and accelerating cell apoptosis [18, 19].
Being in the Arctic region boosts development of hypovitaminosis. Insufficient supply of fat-soluble vitamins can cause severe disorders of the functions of various organs and metabolism in general [16]. Vitamin D3 deficiency translates into disruption of bone mineral metabolism: coupled with lack of essential trace elements, it accelerates the said metabolism and raises the risk of osteoporotic fractures [20].
Cold and light stress also alter functioning of the hormonal systems. Almost all cellular functions and physiological systems are synchronized with circadian rhythms, which are governed by external stimuli, light of certain bandwidth and intensity in particular. Seasonal insolation fluctuations and insufficient UV radiation amounts in the Arctic cause light stress that contributes to the development of metabolic, immune and mental diseases, hinders wound healing, and weakens body's detoxification capacities [21].
In the Arctic, people actively interact with various mechanisms and machines. The chemicals used in this context can irritate skin and cause allergies. Low temperatures necessitate use of special, often heavy clothing, worn while doing physical work. These factors are also among those that provoke diseases of the musculoskeletal and connective tissues and make their course severe.
Thus, there is a number of diseases that are considerably more common in the Arctic, given its climatic and geographical conditions, than in the milder belt south thereof [6]. Damage to local vessels makes injured tissues hypoxic, regardless of the external conditions. Consequently, tissue hypoxia and ischemic reperfusion syndrome caused by general hypothermia complicates healing of various skin, soft and hard tissues injuries while raising the risk of frostbite. Accumulation of ROS, causing excessive inflammation, also interferes with the healing process. Lack of oxygen and nutrients, the deficiency of which will be aggravated by hypoxia and hypothermia, significantly hinders the processes of tissue regeneration, i.e., synthesis of collagen and angiogenesis [22].
As a rule, people stay/work in the Far North on a rotational basis, which prevents development of persisting adaptive protection mechanisms [19], since it takes a person over 3 years of continuous stay in a given climatic and geographical region to fully adapt [9]. In addition, prolonged stay in the Far North often causes hypovitaminosis, which adversely affects body's regenerative capacities and, simultaneously, raises the risk of infections.
Long time spent away from residential quarters, subsequent severely abridged personal hygiene routines, and prolonged wearing of special protective clothing create the risk of development of inflammatory and infectious diseases of the skin and subcutaneous tissue [23].
In extreme environmental conditions, changes in the circulatory system of the body in general and longer angiogenesis caused by lack of oxygen in particular play one of the key roles in preventing active tissue repair after injury [11]. In this connection, it is feasible to consider damaged tissue revascularization possibilities and methods when developing respective treatment protocols. Restoration of skin and bone tissue strongly depends on the body's ability to form new blood vessels. Angiogenesis ensures cells receive the necessary nutrients and, accordingly, helps to maintain their viability [4]. Blood supply plays a significant part in bone regeneration, as it is a highly vascularized tissue. Paracrine regulation of osteogenesis depends on blood vessels [24]. Thus, technologies and methods that improve angiogenesis can help to significantly accelerate tissue regeneration [4].
Disruptions of the first (inflammatory) phase of the healing process are another problem. In particular, hypothermia delays the release of proinflammatory cytokines, reduces the amount and function of neutrophils, and disturbs chemotaxis of leukocytes and phagocytes [25]. Immunosuppression is one of the negative consequences of hypothermia, combined with delayed healing, increased risk of sepsis, impaired plasma coagulation and platelet function. The latter two factors, in particular, can delay surgical removal of necrotic tissues, which adversely affects the recovery and survival of patients in general [26].
Developing new treatment approaches, medical professionals should prioritize these two key processes prioritized as the most important. However, extreme climate, low population density, remoteness and problematic access to the areas comprising the region affect the setup of the medical care system there, which also conditions rescue of people injured in emergency situations [27]. Therefore, new treatment methods should factor in a considerable delay in delivery of the patient to a qualified medical facility.
New therapeutic approaches to hard and soft tissue damage repair using MMSC
Multipotent mesenchymal stem cells (MMSC) participate in all stages of skin and bone regeneration, so employing them for wound treatment is a promising approach. The key therapeutic effect of MMSC stems from the secretory activity, but they can also differentiate into cells of damaged tissue [28]. The cells regulate the duration of the inflammatory phase, participate in the paracrine regulation of scar formation and reorganization at the later stages. Protocols employing MMSC are particularly relevant for chronic wounds and persisting wounds for which traditional therapies have proven ineffective [29]. Populations of MMSC grown on various bioscaffolds are also widely used for bone tissue regeneration [30]. There is evidence that MMSC can improve the outcomes of thermal damage healing due to their immunomodulatory, antioxidant, and angiogenic effects [31, 32]. The results of the pioneer studies investigating administration of MMSC (injections) to treat injuries sustained under simulated hypoxia and hypothermia suggest a significant expansion of the list of indications for cell therapy [33]. In particular, they were shown to be effective against cold injuries. Injections of MMSC have two key therapeutic effects: alleviation of inflammation and improvement of angiogenesis along wound's periphery [34].
Another developing area of cell therapy is employs MMSC exosomes. These extracellular vesicles contain nucleic acids, proteins and lipids. Proteins, enzymes, and microRNAs contained in exosomes are involved in many biochemical and cellular processes, including inflammation and tissue regeneration. This involvement determines their versatility and the ability to interact with several types of cells and trigger the appropriate cellular reactions. In particular, catalytically active enzymes that promote tissue homeostasis are potentially capable of restoring normal tissue function. This is especially important when an injury or a disease alters its microenvironment and, as a result, disrupts homeostasis and tissue functioning. Compared to most other medicines, such preparations offer a lower risk of overdose or dose insufficiency, since the level of activity of the enzyme is controlled directly by the concentration of the substrate. Thus, the therapeutic effect is proportional to the severity of the injury [35, 36].
The secretion of MMSC contains a wide range of biologically active molecules that can be synthesized with the help of recombinant technologies. For example, growth factors have a significant impact on the restoration of damaged tissues. Vascular endothelial growth factor (VEGF) is involved in angiogenesis and neovascularization, and aids, inter alia, migration of endothelial cells [37, 38]. Fibroblast growth factor (FGF) stimulates production of extracellular matrix in many types of cells participating in wound healing [37, 39]. Platelet-derived growth factor (PDGF) supports wound healing, too; it stimulates proliferation of fibroblasts, smooth muscle cells, osteoblasts and other cells [39]. Growth factors can be used both as a drug per se and as a component of cellular products, with particular purposes of their administration bein tissue engineering and/or cellular reprogramming. They offer efficacy proven in cases of treatment of various injuries, and the technologies of production of proteins in yeast are well-established [40].
The specifics of the effect of the Arctic's climate and geography on the human body increase the urgency of designing medicines and biomedical cellular products that treat the lesion in a complex manner, since tissue hypoxia worsens the wound's condition significantly. Another factor is that none of the currently common approaches is a panacea, as all of them have certain peculiarities (tab. 1). In addition, the use of allogeneic cells, exosomes, or recombinant growth factors suffers several significant limitations, both therapeutic and technological.
In this connection, one of the prioritized task is selection and development of a biological matrix for the used cells, exosomes, or proteins. Currently, autografts or cell-free allografts are the golden standards in bone restoration and skin damage treatment, because scaffoldings used in the respective protocols are fully compatible from the physico-chemical and biochemical viewpoints, they ensure the degree of porosity needed for angiogenesis and offer the required proliferative and osteoinductive capacities. Small area of donor sites and high risks of scarring underpin development of full-size skin equivalents and osteoinductive materials [41–43]. Drugs, surgical methods, and medical devices available today cannot fully substitute for all the functions of damaged tissue or a lost organ [44] because of the importance of extracellular matrix: it provides a physical basis for maintaining the integrity of tissues and organs, and also serves as an inducer of biochemical and biophysical signals. This matrix creates a microenvironment that includes highly complex cellular interactions and molecular genetic processes [45]. Tissue engineering can solve the respective problems.
As a base, the matrix can have both natural materials (preferred solution) and synthetic polymers with finely adjustable physico-chemical properties. Table tab. 2 presents the required characteristics of bioscaffolds.
Collagen, which is the key component of extracellular matrix of most body tissues, remains the preferred choice among natural polymers. Its derivative, gelatin, is also widely used in wound dressings designs. Hyaluronic acid, common in our tissues, is another natural polymer suitable for the development of scaffolds supporting restoration of bone and soft tissues. The main advantage of these polymers is their affinity to the human extracellular matrix, but the associated high cost, risks of disease transfer, insufficient supply of raw materials, and other factors necessitate search for other polymers [44]. Scaffolds based on fibrin, silk, chitosan, agarose, cellulose, starch, alginate, and synthetic polymers are also being developed for skin restoration purposes. As for bone tissue engineering, osteoinductivity, an important property of the material, limits its potential considerably, while skin tissue engineering does not have such limitations. Nevertheless, not only collagen, fibrin and hyaluronic can underpin bone scaffolds, but also chitosan, which supports attachment and proliferation of osteoblast bone-forming cells and ensures formation of a mineralized bone matrix [46].
The conditions of the Arctic disrupt tissue trophism, which renders body's own reserves insufficient for repair of the related tissue damage. This fact substantiates the need for new, most effective therapy. One of the possible solutions revolves around matrices containing MMSC, exosomes, or recombinant growth factors; such preparations reduce inflammation and enhance angiogenesis in the first place.
CONCLUSION
The effect of the Far North's natural and climatic conditions is multifactorial, but it does not render impossible development of pluripotent therapeutic products and methods based, in particular, on multipotent mesenchymal stem cells. The said products and methods can be used to treat skin and tissue injuries in other climatic zones, and also help repair damage sustained under extreme conditions of different nature, for example, in the context of a spaceflight, high altitude activities, scuba diving.