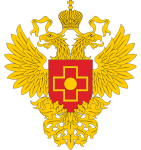
ORIGINAL RESEARCH
N protein based vaccine against SARS-CoV-2 produces a strong T cell immune response to N Protein of novel strains
1 Saint Petersburg Scientific Research Institute of Vaccines and Serums and Enterprise for the Production of Bacterial Preparations of the Federal Medical Biological Agency, Saint Petersburg, Russia
2 Centre for Strategic Planning and Management of Biomedical Health Risks of the Federal Medical Biological Agency, Moscow, Russia
3 Institute of Immunology of the Federal Medical Biological Agency, Moscow, Russia
4 Pirogov Russian National Research Medical University, Moscow, Russia
5 Federal Medical Biological Agency, Moscow, Russia
Correspondence should be addressed: Sevastyan O. Rabdano
Svobody, 52, 198320, Krasnoye Selo, Saint Petersburg; ur.onadbar@naytsaves
Author contribution: Rabdano SO — study design, data analysis, data interpretation, manuscript writing; Mukhin VE — bioinformatic analysis, experimental procedure, data acquisition, statistical analysis, manuscript writing; Makarov VV — study design, data interpretation, manuscript writing; Rudakov GO — data analysis, statistical analysis, data interpretation, graphics preparation, manuscript writing; Ruzanova EA, Arakelov SA, Khaitov MR, Yudin SM, Kryuchko DS, Berzin IA, Evtushenko AE — study design, manuscript editing; Truhin VP, Skvortsova VI — research idea, study concept, manuscript editing.
Compliance with ethical standards: the study was approved by the Ministry of Health of the Russian Federation (clinical trial approval № 388 of 19 July 2021), Ethics Committee of MH RF (protocol № 282 of 19 July 2021) and Independent Ethics Committee (IEC) of the research center (protocols № 163 of 15 July 2021 and № 164 of 20 July 2021); the study was conducted in accordance with the principles of the World Medical Association (WMA) Declaration of Helsinki (1964) and its latest update (2013), tripartite guideline for Good Clinical Practice approved by the International Council for Harmonisation of Technical Requirements for Pharmaceuticals for Human Use (ICH) (E6 (R2) of 09 November 2016) and current legislation of the EEU and RF. Two copies of the informed consent form (volunteer information sheet) were to be signed and dated by the subjects and the researcher by their own handwriting.
The COVID-19 pandemic has been a global challenge since 2020. High infectivity of SARS-CoV-2, high morbidity and mortality [1, 2], as well as rapid virus evolution [3] are the main features of the pandemic. More than 600.5 million cases of COVID-19 and more than 6.5 million deaths were officially reported by September 2022 [4]. Additionally, even non-fatal cases of the disease can have long-term effects on human health, especially on the cardiovascular system [5–7]. The development of universal COVID-19 vaccine capable of producing long-lasting immunity against common viral strains is therefore an urgent task for world pharmaceutical industry.
It is difficult to develop such a vaccine due to high antigenic variability of the virus [8], including the viral spike (S) protein [9]. Today, all of the certified and widely used vaccines are based on the S protein [10]. High specificity of antibodies and T-cell receptors, which manage the humoral and cell-mediated immune responses to the virus, combined with its fast evolution causes the efficiency of both virus neutralization and elimination of infected cells to decrease over time. Past studies present data on the reduction of neutralization of new viral strains by sera of individuals vaccinated with S proteinbased vaccines [11, 12]. There are also many publications describing the reduced efficiency of viral epitope recognition by T-cells, and therefore T-cell activation by protein epitopes of new viral strains [11, 13–16]. However, there are controversial studies describing the persistence of T-cell immune response to peptides containing mutations typical for new viral variants [17].
Overall, the effectiveness of existing S protein-based COVID-19 vaccines decreases over time due to evolution of the virus [18]. As such, the conserved nucleocapsid (N) protein, which is less susceptible to mutation, is a much better target for the formation of long-lasting immunity against the virus than the S protein [19–21]. The protective potential of vaccines based on viral nucleocapsid proteins was demonstrated for influenza [22–26], dengue [27–29] and coronaviruses [20, 30]. An immune response targeted against viral nucleocapsid proteins allows T-cells and antibody-mediated natural killer cells to rapidly destroy infected cells and ensure complete elimination of the virus from the body. Furthermore, the conserved nature of N protein entails its high similarity in different stains, which results in the capability of N-protein-based vaccines to provide protection against a wide range of SARS-CoV-2 variants.
The Saint Petersburg Scientific Research Institute of Vaccines and Serums has developed Convacell®, an N protein-based vaccine against SARS-CoV-2. The amino acid sequence of the antigen in the vaccine corresponds to the N protein of the Wuhan variant. The vaccine is currently undergoing combined phase I and II clinical trials. Due to its target selection, the Convacell® vaccine should produce a long-lasting immunity against all variants of SARS-CoV-2. This advantageously distinguishes it from the currently available S protein-based vaccines. This study was aimed to demonstrate the capability of Convacell® to evoke specific immune response to a range of currently existing SARS-CoV-2 strains.
METHODS
Laboratory studies were performed in the Department of Analysis and Forecasting of Medical and Biological Health Risks, Centre for Strategic Planning and Management of Biomedical Health Risks of FMBA of Russia. A total of 12 subjects were enrolled. Convalescents: six patients with the diagnosis of COVID-19 confirmed by laboratory tests, who had a mild disease in January 2022 (three men and three women aged 18–51). Vaccinated individuals: volunteers fully vaccinated with Convacell® (two doses with an interval of 21 days), the other data on vaccinated subjects have been blinded until the end of the clinical trial. The inclusion/exclusion criteria and other details of the studies are provided in the ClinicalTrials.gov database (NCT05156723).
The convalescents' blood was collected on the day of recovery, and blood of vaccinated individuals was collected on day 42 after administration of the first dose. Peripheral blood samples for laboratory testing were collected by venipuncture of a cubital vein in the morning in the fasting state (at least 8 hrs after the last meal). Blood was collected in sterile vacuum tubes containing K3-EDTA as anticoagulant.
Peripheral blood mononuclear cells (PBMCs) were obtained from peripheral blood samples. The peripheral blood sample volume was at least 6 ml. All the procedures were performed not later than 8 hrs after the collection of biomaterial. The samples were stored and transported at room temperature (18–25 °C) while maintaining the cold chain.
PBMCs were isolated from peripheral blood by the Ficoll density gradient centrifugation (1.077 g/l). Whole blood was gently mixed several times by inverting the tube 5–6 times by 180°, diluted in Dulbecco's phosphate buffered saline (DPBS) to obtain a 1:1 dilution, and then layered onto a Ficoll-based medium with the density of 1.077 g/mL and centrifuged at 450 g for 30 min with disabled rotor brake at room temperature. After the end of centrifugation, the interphase ring located on the boundary between the Ficoll-based medium and blood plasma was harvested with a serological pipette and transferred to a clean 15 ml conical tube. Then the cells were twice washed with DPBS by centrifugation at 300 g for 10 min (Eppendorf; Germany). After the second washing, cells were enumerated, and cell viability was assessed by trypan blue staining with the Countess 3 Cell Counter (Thermo; USA). The cells were subsequently diluted with RPMI 1640 complete medium (10% fetal bovine serum, 1% penicillin-streptomycin) to a concentration of 106 cells/mL. Cell viability was at least 95%.
The quantity and phenotypes of antigen-specific cells were defined by multicolor flow cytometry based on the cell capability to produce cytokines in response to stimulation with peptides covering N protein regions of various SARS-CoV-2 variants. 106 PBMCs in 100 µl of culture medium were introduced to flow cytometry tubes, supplemented with 1 µg/ml of antigen (protein or pooled peptides) and incubated for 12 hrs at 37 °С in 5% CO2. Two hours after adding antigen, 10 µg/ml of brefeldin A were added to the cells (Sigma-Aldrich; USA). After the end of incubation cells were washed with DPBS, then cell surface was stained with the anti-CD3 (UCHT1), anti-CD4 (13B8.2), anti-CD8 (B9.11), anti-CD45RA (2H4), anti-CD197 (G043H7) antibody conjugates (all antibodies manufactured by Beckman Coulter; USA). Zombie Aqua™ Fixable Viability Kit (Biolegend; USA) was used to assess cell viability. Then, cells were washed with DPBS and fixed, cell membranes were permeabilized using the IntraPrep Permeabilization Reagent Kit (Beckman Coulter; USA), and intracellular staining was performed using the anti-IL2 (IL2.39.1) and anti-IFNg (45.15) antibody conjugates (Beckman Coulter; USA).
The CytoFlex flow cytometer (Beckman Coulter; USA) was used for analysis. At least 100,000 T-cells per sample were recorded. The results were analyzed with the CytExpert Acquisition and Analysis Software, ver. 2.4 (Beckman Coulter; USA), plots were prepared using the GraphPad Prism 9 software. Analysis of variance (ANOVA) was used for statistical analysis in all cases.
RESULTS
Cytometry of PBMCs stimulated by N proteins of the Wuhan, Delta and Omicron strains
Simulation of the contact between the immune cells obtained from vaccinated individuals and convalescents and the Wuhan, Delta and Omicron strains of SARS-CoV-2 was done by incubation of the cells after adding the representative antigens of each strain. The cells were incubated with the following three variants of the SARS-CoV-2 antigen: а) full-length N protein with the sequence corresponding to the that of the reference strain (Wuhan); b) Delta strain N protein peptide pool; c) Omicron strain N protein peptide pool.
The following analysis was performed to provide the Delta and Omicron strain peptide pools.
Mutations in the nucleocapsid protein of the virus of the clades we were interested in were selected in the Lineages/ Mutations section of the Coronavirus 3D website [31].
The reference nucleotide sequence of N protein (Wuhan strain) and full-genome reference sequence for the Delta B.1.617.2 strain (EPI_ISL_1663516) were downloaded from the GISAID website [32]. Currently there are no reference full-genome sequences for the strains Omicron BA.2, BA.4, BA.5 available from the GISAID database.
N protein nucleotide sequences were translated into amino acid sequences with the VIGOR4 tool [33].
Mutations selected in paragraph 1 were incorporated into the reference N protein sequence using UGENE [34]. Four resulting FASTA files were integrated into one multi-FASTA file.
Multiple sequence alignment was performed using the MUSCLE tool [35].
The alignment of N protein sequences typical for various SARS-CoV-2 strains (fig. 1) showed that the differences between the nucleocapsid proteins of the Delta and Omicron strains could be limited to the listed below mutations, compared to the Wuhan reference strain N protein. Omicron: P13L, 31-33del, P151S, R203K, G204R, S413R; Delta: D63G, R203M, G215C, D377Y.
Peptide pools with five mutations, three peptides per mutation and 11 overlapping amino acids were selected based on the analysis to represent each strain (fig. 2). Despite the availability of data on the immunodominant epitopes in vaccinated individuals and COVID-19 convalescents [36], we chose epitopes containing mutations, since these were the most representative in terms of changes in the immune response due to virus evolution.
To assess and compare cell-mediated immune responses evoked by the Convacell® vaccine and SARS-CoV-2 infection, blood was collected from participants of the Convacell® vaccine clinical trials and volunteers who survived COVID-19. Then, PBMCs were isolated and frozen until the analysis was performed. After thawing, cells were divided into aliquots and subjected to specific stimulation with antigens of the Wuhan, Delta and Omicron strains. Furthermore, the corresponding negative (culture medium) and positive (phorbol myristate acetate/ionomycin) controls were introduced. The details of stimulation protocol are provided in the Methods section.
After stimulation, PBMCs were stained with fluorescent antibodies against markers corresponding to the major T cell phenotypes (CD4, CD8) and markers of their activation (IFNγ, IL2). Then, cytometry analysis of the stained PBMCs was performed.
The results of the analysis (fig. 3) showed that for samples from vaccinated individuals the stimulation with N protein generally evoked similar immune responses in all the studied subpopulations of T-helper cells and killer T cells for all strains of SARS-CoV-2.
CD4+INFγ+ PBMC subpopulation: vaccination with Convacell® evoked the response similar to that produced due to infection; no significant differences in the number of activated cells between N proteins of various strains were found in both vaccinated individuals and convalescents.
CD8+INFγ+ PBMC subpopulation: a slightly stronger immune response was observed in convalescents compared to vaccinated individuals; stimulation with N proteins of the Wuhan and Delta strains also evoked stronger immune response than stimulation with Omicron N protein. However, no significant differences were revealed. N protein stimulation produced strong immune response in all cases.
CD4+IL2+ cells: strong immune response was produced in vaccinated individuals, and a significantly weaker response was observed in convalescents. No differences were revealed during stimulation with N proteins of various strains.
CD8+IL2+ cells: equally weak responses were observed in all subpopulations, regardless of the cell phenotype and protein strain used for stimulation.
Phenotyping of memory cells
Further analysis of the phenotype of specific memory cells (fig. 4) that were stimulated in samples from individuals that were vaccinated with Convacell® and COVID-19 convalescents after stimulation with N proteins of various viral strains revealed no differences in the structure of immune response in all populations.
Predominance of effector memory (EM) T cells at the level of about 60% of all activated cells and prevalence of central memory (CM) T cells at the level of about 30% of the total cell number are typical for T-helper cells. Naive Т cells constitute about 10%, while TEMRA cells are rare. There are no significant differences between the convalescents and vaccinated individuals, as well as between the SARS-CoV-2 strains.
Cytotoxic T-cells are characterized by the presence of effector memory T-cells at 40–50% of total number and TEMRA cells at 20–40% of total number. Naive Т cells and central memory T cells constitute 10–20% of total number. Stimulation with the N protein of Omicron strain results in higher proportion of TEMRA cells and lower proportion of naive T-cells and central memory T-cells compared to stimulation with the Delta strain protein, however, the differences are non-significant. There are no differences between the convalescents and vaccinated individuals.
DISCUSSION
Studies assessing the proportion of CD4 and CD8 cells expressing IFNγ in response to stimulation with antigens were also published earlier [17, 37]. In individuals vaccinated with mRNA vaccines and S protein from homologous variant, the immune response was stronger than in COVID-19 convalescents [17]. A stronger T-cell response, in particular CD4+IL2+ cells (see comparison of gray and red bars in fig. 3), is observed for vaccinated with Convacell® compared to convalescent patients, however, unlike S-protein vaccines, this effect is spread and non-homologous strains. In blood samples obtained from convalescents, about 40 of 105 CD4+ cells and 50 of 105 CD8+ cells express IFNγ when stimulated with N protein peptides [17]. Our findings show that the number of cells responsive to stimulation in convalescents is slightly lower than the number reported in the paper [17]. It should be noted that the number of T cells responsive to stimulation with S protein peptides rapidly decreases in both convalescents and individuals vaccinated with mRNA vaccines [37, 38]. The number of cells decreases by a factor of two within 1–1.5 months on average.
The findings show that the Convacell® vaccine is capable of evoking the same or even stronger immune response as the disease: there are no significant differences in the number of activated cytotoxic T cells and T helper cells between the vaccinated subjects and convalescents, and the number of T helper cells producing IL2 is significantly higher among vaccinated individuals. The data obtained confirm Convacell®’s capability to produce highly potent cell-mediated immune response to the SARS-CoV-2 N protein.
There are also no significant differences in the number of activated cells in all the vaccinated populations when stimulated with N protein of any of the viral strains used. This demonstrates Convacell® capability to produce equally strong immunity against new strains.
Phenotyping of CD4 memory cells in vaccinated individuals and convalescents was also conducted earlier [37]. The CD4+-CM and CD4+-EM cell predominance (40–50% of all responsive cells) revealed in our study is consistent with the data provided by these studies.
The lack of differences in the structure of immune response in vaccinated individuals stimulated with proteins of various SARS-CoV-2 strains confirms Convacell® capability to produce equally strong cell-mediated immune response against all viral strains. Similarities in the structure of immune responses resulting from both vaccination and disease testify in favor of the efficiency of the immune response produced by the vaccine.
CONCLUSIONS
The Convacell® vaccine showed capability to produce equally strong cell-mediated immune response against the SARS-CoV-2 Wuhan, Delta and Omicron strains. This emphasizes the potential of the N-protein-based approach to the development of universal COVID-19 vaccine. Our study demonstrates Convacell®’s capability to produce highly potent cell-mediated immune response against the currently circulating SARS-CoV-2 strains. In view of rapid virus evolution, availability of universal vaccine would ensure protection of the population against new waves of COVID-19.