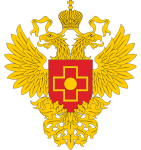
ORIGINAL RESEARCH
Fabrication of cartilage tissue substitutes from cells with induced pluripotency
1 Federal Research and Clinical Center of Physical-Chemical Medicine of Federal Medical Biological Agency, Moscow, Russia
2 Lomonosov Moscow State University, Moscow, Russia
3 Koltzov Institute of Developmental Biology, Moscow, Russia
Correspondence should be addressed: Artem V. Eremeev
Malaya Pirogovskaya, 1a, 119435, Moscow, Russia; ur.xednay@veemere-tra
Funding: the study was supported with an allocation #22-15-00250 by the Russian Science Foundation.
Acknowledgments: the authors thank Corresponding Member of the Russian Academy of Sciences M.A. Lagarkova for providing research facilities for the study
Author contribution: Eremeev AV — design of the experiment, general guidance, article authoring; Pikina AS — literature review, collection of the material, participation in the experimental part of the work, analysis of the resulting data; Ruchko ES — participation in the experimental part of the work; Sidorov VS, Ragozin AO — provision of material for the experiment.
Compliance with ethical standards: the study was performed in conformity with the principles of the Declaration of Helsinki (2000) and its subsequent revisions.
Peculiarities of morphology of the hyaline cartilage tissue make its ability to heal rather low. Without proper therapy, most defects of the cartilage caused by trauma, focal lesions or degeneration processes progress, for example, into arthrosis, which negatively affects the quality of life. Cellular technologies that can cover losses of functionally active cells in the damaged tissue area and trigger effective healing offer a promising approach to this problem.
CO.DON, a German company, has been using such cellular technologies in the clinic for over 10 years: the respective protocols of treatment of the damaged articular cartilage rely on autologous chondrocytes, which ensure development of a phenotypically stable cartilage during healing [1–3]. However, transplantation of autologous chondrocytes, although a proven successful approach to the damaged hyaline cartilage restoration, is quite invasive: collection of the donor material requires a biopsy [4, 5]. In addition, the amount of cellular material collected is quite small, which necessitates long-term cultivation that puts the cells at risk of losing chondrogenic qualities and differentiating into fibroblasts, which can lead to fibrosis after transplantation [4, 5]. In connection with these shortcomings, it is especially important to have a selection of alternative cellular resources.
Induced pluripotent stem cells (iPSCs) are one of the promising sources of cellular material. Their properties, such as pluripotency, a wide potential for differentiation into all types of somatic cells, including chondrocytes, as well as the unlimited self-renewal ability, make iPSCs an equivalent of embryonic stem cells (ESCs) that lacks the ethical problems associated with derivation of the latter [4, 6, 7]. Any type of somatic cells of the body can be used to make iPSCs [5, 8].
Chondrocytes differentiated from iPSCs have a juvenile phenotype, which translates into a high proliferation rate and increased production of extracellular matrix (ECM). This quality makes healing of articular defects more effective [4, 5]. Thus, iPSCs are a promising source of cells that can yield a large amount of biomaterial for cellular technologies. However, despite a large number of studies investigating the subject, there is still no standardized protocol that ensures quality chondrogenic differentiation [9]. An actively used method is that of directed differentiation, which roughly reproduces the process of chondrogenesis [10]. The common choice in this context are recombinant proteins that are similar to the main chondroinducers in the developmental processes, as well as various combinations thereof (fig. 1).
Proteins of the transforming growth factor — (TGFβ) superfamily, such as TGFβ proper and those of the bone morphogenetic protein family (BMP2), are widely used for in vitro chondrogenic differentiation. One study [11] had TGFβ3 as the only differentiation factor, and the resulting chondrogenesis in iPSC cultures was registered as incomplete. Another group of researchers relied on a combination of TGFβ1 and BMP2 [12], and the results they achieved were of better quality. The effectiveness of in vitro chondrogenesis may also be increased through differentiation into an MSC-like cell population as a preparative step; this approach was used in the "classic" protocol [3], which implies preliminary induction of mesenchymal precursors with Wnt3a and Activin A. The next step was to induce chondrogenesis using TGFβ1 and BMP2, thus creating cartilage structures with a high expression of chondrogenic markers and a low level of hypertrophy. A similar protocol that differed in longer cultivation time has also yielded effective chondrogenesis; the resulting structure was transplanted subcutaneously to a mouse with subsequent formation of a cartilage of a juvenile phenotype with a high content of proteoglycans [4]. Retinoic acid (RA) and retinoids are necessary for the development of limbs, since they trigger activation of Hox genes involved in determining the area of bud formation [13]. In vitro, a combination of Chir 99021 (6-[[2-[[4-(2,4-dichlorophenyl)-5-(5-methyl-1H-imidazol-2-yl)-2pyrimidinyl]amino]ethyl]amino]-3-pyridinecarbonitrile, an inhibitor of glycogen synthase kinase 3 (GSK-3)) and RA promoted directed differentiation into chondrocytes within a short period of time [14]. In addition, low molecular weight compounds are quite simple to use, non-immunogenic and can be efficiently delivered to cells [15].
The standard 2D culture approach does not match the natural environment of the cells and limits differentiation significantly [16]. Both in vivo and in vitro, cells need a 3D environment.
One of the common methods of 3D cell cultivation and differentiation is the production of spheroids [17, 18]. Spheroid cultures were shown to significantly improve cell proliferation while maintaining the phenotype and key signals [16, 19]. Moreover, this 3D culturing technique mimics the process of mesenchymal condensation at the early stage of cartilage development [20]. There are various methods yielding spheroids, including the hanging drop method [21, 22], centrifugation of a suspension of cells of certain density [23–25], self-aggregation into spheroids in suspension cultures [2], formation in U-shaped microwell plates [26–28], as well as methods involving biomaterials [29, 30]. The finished constructs can be effectively cultured under dynamic conditions, for example, in a 3D orbital shaker [8].
In this work, we followed four protocols of the 3D spheroid culturing method to form cartilage tissue. Two of the four protocols were developed by our laboratory. The main purpose of this study was to identify and compare the features of the resulting structures and single out the most effective way of differentiation.
METHODS
iPSC cultures
We used the FD4S iPSC line derived from human skin fibroblasts by the method described in [41], using a non-integrating Sendai viral vector carrying the genes of transcription factors OCT3/4, SOX2, KLF4, and C-MYC. The cells were cryopreserved at –80 °C.
Cultivation was conducted at 37 °C with CO2 at 5%, in a mixture of growth media without a mTeSR1 feeder (STEMCELL Technologies; Canada) and Essential 8 (Thermo Fisher Scientific; USA), at a 1 : 3 ratio, with 40 µg/ml of gentamicin (PanEco; Russia). The medium was changed once a day. Upon appearance of a monolayer, we subcultured the culture at 1 : 3 ratio; to improve cell viability after this procedure, we used 10 µM of the Y27632 Rock kinase inhibitor (StemMACS, Miltenyi Biotec; Germany).
Differentiation protocols
Chondrogenic differentiation of iPSCs was conducted following the four tested protocols (tab. 1):
Cultures of human chondrocytes and fragments of human articular cartilage were used as a positive control. Cultures of iPSCs and 3D structures from them served as negative control.
Monolayer cultures
Monolayer iPSC cultures were differentiated for 7 days in Advanced DMEM base medium (Gibco, Thermo Fisher Scientific; USA) supplemented with 10 ng/ml of bFGF (STEMCELL Technologies; Canada), 100× GlutaMAX (Gibco, Thermo Fisher Scientific; USA), 50x B27 (Gibco, Thermo Fisher Scientific; USA), 1% insulin transferrin selenite (ITS) (PanEco; Russia), 50 µg/ml ascorbic acid (Sigma Aldrich; USA), 50 µM β-mercaptoethanol, 5 µg/ml plasmacin, gentamicin (PanEco; Russia) and 40 µg/ml gentamicin solution (PanEco; Russia).
For the "long" protocol we also added 10 ng/ml of TGFβ1 (Miltenyi Biotec; Germany), 10 ng/ml of BMP2 and 10% FBS to the base medium. For the "short", "combined" and conditioned medium protocols the supplements were 10 µM of Chir 99021 (Miltenyi Biotec; Germany) and 10 nM of RA (Sigma Aldrich; USA) introduced together for two days, and after that — only 10 nM of RA.
The medium was changed once a day. On the third or fourth day we subcultured the cultures at the ratio of 1:3 with a 0.25% trypsin solution.
The previously obtained culture of human chondrocytes was thawed from the cryobank of the Federal Research And Clinical Center of Physical-Chemical Medicine. The process implied heating the cryovial in a water bath until the ice completely disappeared and then washing the DMSO cryoprotector off the cells in 10 ml of pure Advanced DMEM medium preheated to +37 °C, the washing done by centrifuging in a 15 ml test tube (Servicebio; China) at 1000 RPM for 5 min. The precipitate with chondrocytes was diluted for subsequent cultivation in Advanced DMEM supplemented with 15% FBS or 10% human serum. The medium was changed every 4 days; the conditioned medium was taken out and filtered twice through syringe filters (0.45 µm pore and 0.22 µm pore, respectively).
3D cultures
The spheroids were formed in AggreWell 800 microwell plates (STEMCELL Technologies; Canada) with Anti-Adherence Rinsing Solution (STEMCELL Technologies; Canada); we followed the protocol provided by the manufacturer [31].
Factoring in the number of cells, we added 1 or 2 ml of medium with 10 µM Y27632, as per the protocol of chondrogenic differentiation in 3D cultures, seeking to reach the concentration of 1–1.5 × 106 cells/ml. Each well of a plate contained 1 ml of this suspension. The plates with cells evenly distributed in microwells were incubated at 37°C with CO2 at 5% for 24 h.
To prepare Petri dishes for cultivation of spheroids we applied chloroform-plastic glue strictly to the center of 60 mm Ultra Low Attachment Petri dishes (Corning Inc.; USA). The applied chloroform-plastic glue had the shape of a drop with the diameter of about 1 cm. Then, the cups without lids were placed under ultraviolet light for 6 hours. Before use, we rinsed the surface several times with Versene solution [32].
After 24 h of incubation in microwell plates, we carefully collected spherical cell aggregates using pipettes with tips cut off (to avoid damage to the spheroids) and then transferred them to prepared Petri dishes, the medium therein as per the differentiation protocol. Dishes with spheroids were subjected to dynamic processing in a 3D orbital shaker at 37°C and with CO2 at 5%.
For the differentiation of 3D cultures under the "long", "short" and "combined" protocols, as well as to cultivate the positive control spheroids, we used the Advanced DMEM base medium supplemented with 10 ng/ml of bFGF (STEMCELL Technologies; Canada), 100× GlutaMAX (Gibco , Thermo Fisher Scientific; USA), 50× B27 (Gibco, Thermo Fisher Scientific; USA), 1% insulintransferrin selenite (ITS) (PanEco; Russia), 50 µg/ml of ascorbic acid (Sigma Aldrich; USA ), 50 µM of β-mercaptoethanol, 5 µg/ml of plasmacin, gentamicin (PanEco; Russia) and 10 ml/L 100x penicillin/streptomycin solution (PanEco; Russia). For the "long" and "combined" protocols we also added 10 ng/ml of TGFβ1 (Miltenyi Biotec; Germany), 10 ng/ml of BMP2, 10% FBS to the medium. For the conditioned medium protocol we used the conditioned medium from a culture of human articular chondrocytes supplemented with 200x GlutaMAX. For cultivation of the positive control spheroids the medium was supplemented with 10% FBS. Negative control spheroids were cultured in Advanced DMEM supplemented with antibiotics and 200x GlutaMAX.
The period of differentiation of spheroids was 28 days. The medium was changed every 4 days. We evaluated morphology of the spheroids every 7 days using an Olympus IX53F phase contrast microscope (Olympus; Japan) and CellSens Standart morphometry software.
Immunofluorescence analysis
For the purposes of immunocytochemical staining of 3D cultures, every 7 days of cultivation we transferred the spheroids into 48-well plates that had bottoms of wells preliminarily covered with a 0.1% gelatin solution. Within 1 to 2 days, the spheroids attached and spread over the surface.
Monolayer cultures fixed with 4% paraformaldehyde (PFA) and attached organelles were treated with 0.1% Triton as follows: for 20 minutes in order to stain for the nuclear marker; for 10 minutes — to stain for the surface and cytoplasmic markers. After permeabilization, the cultures were treated (for 30 minutes) with a 0.01 M PBS blocking solution containing 3% goat serum and 0.1% Tween.
Monolayer cultures, as well as spheroids at all stages of differentiation, were stained with primary antibodies to the nuclear marker of chondrogenesis Sox 9 (Rabbit, 1 : 400, Invitrogen; Thermo Fisher Scientific, USA), to the proteoglycan cartilage ECM marker agrecan (Mouse, 1 : 500, Invitrogen; Thermo Fisher Scientific, USA), fibrillar ECM hyaline cartilage marker type II collagen (Rabbit, 1 : 200; Abcam, UK) and fibrocartilage marker type I collagen (Rabbit, 1 : 800, Invitrogen; Thermo Fisher Scientific, USA), as well as the surface marker of CD105 prechondrogenic mesenchyme (Human, 1 : 500; Sony, Japan). At room temperature, the staining with primary antibody solutions based on blocking solution lasted for 1.5 hours, while at 4 °C the duration thereof was 12 hours.
Alexa Fluor 488 (Goat, Anti-Mouse, 1 : 500), Alexa Fluor 555 (Goat, Anti-Rabbit, 1 : 500), and Alexa Fluor 546 (Goat, Anti-Human, 1 : 500) were used for staining with secondary antibodies (Invitrogen; Thermo Fisher Scientific, USA). The process lasted 1 hour and was conducted in the dark. Nuclei were stained with 100 ng/ml DAPI (Sigma Aldrich; USA).
Stained preparations were examined using an Olympus IX53F fluorescence microscope with four fluorescence filters (Olympus; Japan) and CellSens Standard morphometry software.
Real-time polymerase chain reaction (PCR)
To initiate cell lysis in monolayer cultures and spheroids we used RLT buffer (QIAGEN; Germany) supplemented with 10 µl/ml of β-mercaptoethanol. Spheroids, in batches of 3 to 5 pieces, depending on their size, and monolayer cultures were pipetted into 600 µl of RLT for lysing.
To isolate the total RNA, we used RNeasy Plus Mini Kit (QIAGEN; Germany) following the instructions supplied therewith [33]. Total RNA was purified from genomic DNA with the help of DNase solution (SibEnzyme; Russia) MMLV RT kit (Evrogen; Russia) was used, as described in the manual [34], to synthesize the first cDNA strand from the RNA template.
For real-time PCR, we added 5 µl of 5× qPCRmix-HS SYBR (Evrogen; Russia), 0.8 µl of 10 µM primer, 18.2 µl of water and 1 µl of cDNA matrix (tab. 2) to each well of a 96-well plate (SSIbio, Scientific Specialties; USA), . The reaction was enabled by a C10000 Touch version of 1000 CFX Manager nucleic acid amplification thermal cycler (Bio-Rad; USA) and CFX Manager software. The number of cycles was 39. SYBR (Evrogen; Russia) was used as the probe. To increase specificity of the reaction we used a "hot start" polymerase, HS Taq DNA polymerase (Evrogen; Russia), and selected the optimal primer annealing temperature (60 °C). cDNA isolated from iPSCs was used as a negative control in assessment of specificity of the reaction once the results were available.
Microsoft Excel enabled analysis of the results (ΔΔCt method). Mean values and confidence intervals are shown. For statistical analysis, we used the Welch's t-test that accounts for the possible differences in standard deviations of means of two groups of independent samples.
Histological analysis
To make paraffin sections, we sequentially fixed spheroids and fragments of cartilage, treated them with xylene and ethanol (concentrations of 70, 80, 96, and 100%) to dehydrate and degrease, and poured liquid paraffin. Then we cut series of paraffin sections 4 µm thick. Cryosections of spheroids (7 µm thick) were prepared according to the protocol described earlier [35]. For that purpose, we used the Shandon Cryotome FSE resin (Thermo Fisher Scientific; USA) to form a histological block. The sections were stained with hematoxylin-eosin, picrosirius red, and safranin O. After staining they were dehydrated and embedded in polystyrene.
The photographed of the sections were taken with a DM4000 B LED microscope (Leica; Germany).
RESULTS
Differentiation in monolayer cultures
Undifferentiated iPSC cultures were dense colonies of small cells with a high nuclear-cytoplasmic ratio; the morphology of such colonies was described earlier [36]. On the second day of differentiation by exposure to Chir 99021 and RA or recombinant factors TGFβ1, BMP2, and 10% FBS, the cells assumed a rounded shape. Following the Chir 99021 differentiation protocols, we registered growth in cell mortality, which was assessed by staining with a trypan blue solution (the volume of dead cells that included the dye reached 30–35% of the population). On the 4th day of differentiation the cells assumed a polygonal and elongated shape. On the 7th day we noticed individual populations of chondrocyte-like cells that had a rounded shape and a large nucleus. Also, on the second and third day of differentiation we observed budding cell clusters in the experimental group cultures, the effect especially pronounced in cultures differentiated according to the Chir 99021 protocol.
Analyzing the results of immunocytochemistry we discovered a significant fluorescence on the part of aggrecan and type I and II collagens in monolayer cultures obtained following protocols implying the use of both recombinant factors TGFβ1 and BMP2 and Chir 99021 and RA (fig. 2). However, the Sox9 synthesis was most effective in cultures differentiated with the help of TGFβ1 and BMP2 (fig. 2A1). As for the CD105 mesodermal marker, it synthesis was low in experimental and control groups (fig. 2A4–E4), although a small signal was observed in cultures differentiated with Chir 99021 (fig. 2B4).
The analysis of results of real-time PCR revealed that the indicators of expression of chondrogenic markers in the samples were comparable to those registered in the positive control group. The use of protein factors TGFβ1 and BMP2 made the expression of SOX9 higher than application of Chir 99021 and RA (fig. 3).
Differentiation in spheroid cultures
Immediately after the formed cell aggregates were put in 3D cultivation conditions they acquired an irregular shape and their surface became uneven, but on the 35th day of differentiation all spheroids (all protocol groups and control groups) became whitish translucent structures with a smooth shiny surface (fig. 1G). The only exception were the cultures developing in 3D cultivation conditions following the conditioned medium protocol.
On the 14th and 21st day of differentiation we observed nonintense fluorescence of aggrecan in the constructs of cultures of spheroids cultivated in 3D conditions, all protocols (A), "short" (B), "combined" (C), with conditioned medium (D). E. Spheroids of the positive control group. F. Spheroids of the negative control group. Duration of differentiation: 1 — 14 days, 2 — 21 days, 3 — 28 days, 4 — 35 days. Scale bar — 200 microns" data-note="" >fig. 4). The highest fluorescence intensity of this marker was recorded on days 28 and 35 in spheroids of the "combined" protocol (A), "short" (B), "combined" (C), with conditioned medium (D). E. Spheroids of the positive control group. F. Spheroids of the negative control group. Duration of differentiation: 1 — 14 days, 2 — 21 days, 3 — 28 days, 4 — 35 days. Scale bar — 200 microns" data-note="" >fig. 4B3, 4). On the 21st and 28th days we also registered a high level of synthesis of aggrecan in spheroids obtained following the conditioned medium protocol (A), "short" (B), "combined" (C), with conditioned medium (D). E. Spheroids of the positive control group. F. Spheroids of the negative control group. Duration of differentiation: 1 — 14 days, 2 — 21 days, 3 — 28 days, 4 — 35 days. Scale bar — 200 microns" data-note="" >fig. 4D1–3). In the "long", "combined" and conditioned medium protocols the level of ACAN expression was comparable to that in the spheroids of the positive control group. At the same time, on the 28th and 35th days of differentiation, ACAN expression significantly increased in the spheroids of "combined" and conditioned medium protocols (fig. 5A).
The synthesis of Sox9 was observed in spheroids formed following the "combined" protocol at each step, and the intensity of fluorescence of this marker was increasing as differentiation progressed (A), "short" (B), "combined" (C), with conditioned medium (D). E. Spheroids of the positive control group. F. Spheroids of the negative control group. Duration of differentiation: 1 — 14 days, 2 — 21 days, 3 — 28 days, 4 — 35 days. Scale bar — 200 microns" data-note="" >fig. 4B1–4). As for other protocols, we registered intense Sox9 fluorescence signals on the 28th day in the "long" protocol spheroids and on the 35th day in the conditioned medium protocol spheroids (A), "short" (B), "combined" (C), with conditioned medium (D). E. Spheroids of the positive control group. F. Spheroids of the negative control group. Duration of differentiation: 1 — 14 days, 2 — 21 days, 3 — 28 days, 4 — 35 days. Scale bar — 200 microns" data-note="" >fig. 4A3, D4). The fluorescence associated with this marker was also seen in spheroids of the negative control group (A), "short" (B), "combined" (C), with conditioned medium (D). E. Spheroids of the positive control group. F. Spheroids of the negative control group. Duration of differentiation: 1 — 14 days, 2 — 21 days, 3 — 28 days, 4 — 35 days. Scale bar — 200 microns" data-note="" >fig. 4E1–4). The results of PCR analysis of SOX9 expression show its comparability with the indicators peculiar to the positive control group in samples of the "long" and "combined" protocol groups (fig. 5B).
The intensity of fluorescence of type I collagen was recorded as high in all experimental groups, but the highest values were registered in the "long" and "combined" protocol groups. The expression of COL1A2 increased with the progress of differentiation in spheroids of all protocol groups (fig. 6A). The highest rates were observed in the "long" and "combined" protocol group samples.
In the spheroids formed following "long", "combined" and conditioned medium protocols the observed intensity of fluorescence of type II collagen was high, and it can be said that it increased with time. The expression of COL2A1 was pronounced in spheroids of all differentiation protocol groups (fig. 6B). The maximum values were registered in the samples of "long" and "combined" protocol groups: they were several times greater than expression seen in the positive control group.
The synthesis of CD105 was detected at the beginning of cultivation under 3D conditions. The fluorescence of this marker was well expressed in the "long" and "combined" protocol spheroids, but it significantly decreased by the end of differentiation.
Examining the sections of "long" and "combined" protocol spheroids stained with picrosirius red we saw bright pink collagen fibers covering the entire area of said sections (A), "short" (B), "combined" (C), with conditioned medium (D). Control groups: positive control (fragments of articular cartilage) (E), negative control (F). Type of histological staining: 1 — hematoxylin-eosin, 2 — picrosirius red, 3 — safranin O" data-note="" >fig. 7). However, staining of spheroids of all protocol groups with safranin O was not intense (A), "short" (B), "combined" (C), with conditioned medium (D). Control groups: positive control (fragments of articular cartilage) (E), negative control (F). Type of histological staining: 1 — hematoxylin-eosin, 2 — picrosirius red, 3 — safranin O" data-note="" >fig. 7).
DISCUSSION
Comparing the morphological characteristics of monolayer cultures, we noted that iPSC differentiation can be induced with both Chir 99021 and RA and TGFβ, BMP2, and 10% FBS. The elongated polygonal shape the cells assume on the 4th day may indicate that their morphology becomes MSC-like during chondrogenic induction. The increased cell death that we observed in cultures differentiated with Chir 99021 and RA is most likely associated with the action of Chir 99021, since, as shown in the past experiments, this molecule enhances apoptotic activity [14]. Self-aggregation of cells with the formation of hyaline-like structures peculiar to the early stages of chondrogenic differentiation, when adhesion molecules actively form and accumulate (10).
The positive effect exogenous presence of TGFβ1 and BMP2 has on the synthesis and expression of Sox9 in monolayer cultures is most likely the result of participation of these molecules in stimulation and stabilization of production of this transcription factor [37]. Since Chir 99021 mimics mesoderm-inducing signals, the presence of CD105 can be explained by similarity of the culture, at that stage of differentiation, to the early prechondrogenic mesenchyme [1, 14]. It can be concluded that use of both TGFβ1, BMP2, and 10% FBS and Chir 99021 and RA triggers fairly efficient differentiation in monolayer cultures.
Microwell plates were used to make spheroids. In such plates, cell aggregation is gravity-driven, which may lead to formation of cell conglomerates of irregular shapes [27]. Cartilage structures that were obtained by other researchers from spheroid cultures were ultimately translucent and whitish, with a smooth surface [3]. At the final stages of cultivation, the spheroids of all protocol groups except for the conditioned medium group has similar characteristics. The exception may be the result of a slower compaction rate, which disallows the outer layer cells to generate a sufficient number of intercellular contact molecules.
In our study, the expression of chondrogenic markers in groups using TGFβ1 and BMP2 were fairly high. In addition, we saw spontaneous differentiation of iPSCs in negative control spheroids, which coincided with registration of fluorescent signals of Sox9 and type II collagen, as well as expression of COL2A1. The production of cartilage ECM is largely a mechanodependent process, therefore, we could observe the synthesis of type II collagen in response to the presence of cells in dynamic conditions [38, 39].
The synthesis of CD105 in spheroid cultures on the 14th and 21st days can be explained by the transition from MSC-like to chondrocyte phenotype, which was happening as cells in spheroids were differentiating. in vivo, CD105, along with other surface markers such as CD34, CD44, and CD45, is one of the markers characteristic of prechondrogenic mesenchymal cells [1, 40].
The increased synthesis and expression of type I collagen in spheroids of all protocol groups indicate that the resulting cartilage structures have a mixed phenotype, combining hyaline and fibrous tissues. The strongest expression of COL1A2 was observed in spheroids formed following the protocols that used 10% FBS. Some studies mention the fibrotic effect of serum associated with the increased synthesis of type I collagen, so it is likely that the high levels of this collagen may be conditioned by its presence in the differentiation medium [41, 42].
Histological analysis revealed no significant presence of the proteoglycan matrix in preparations stained with safranin O. In this connection, it can be assumed that the resulting structures are similar to cartilage at an early stage of chondrogenesis. Probably, generation of a greater amount of proteoglycan matrix that would be detectable with the help of safranin O requires longer culturing. Previous research has shown that on the 28th day of cultivation spheroids could be stained with safranin O marginally, while on the 42th day such staining was intense [3].
CONCLUSIONS
Our study yielded samples of cartilage tissue formed following four protocols. A comparative analysis of these protocols has shown that chondrogenesis is most effective in cultures of 3D spheroids differentiated under a "combined" protocol, which, as we suggest, relies on Chir 99021 and RA for differentiation of monolayer cultures and TGFβ1, BMP2 and 10% FBS for differentiation of spheroid cultures. High rates of synthesis and expression of chondrogenic markers were also registered in constructs obtained following the "long" and conditioned medium protocols. The use of conditioned medium, obtained from the primary culture of chondrocytes from donor tissue and exposed to Chir 99021 and RA, increases the efficacy of differentiation. In our opinion, use of a conditioned medium reduces the cost of the technology, but complicates its standardization the because of the variability of different cultures of chondrocytes obtained from different donors. This problem can be solved by using cultures of chondrocytes derived iPSCs. This protocol needs further optimization, as it enables formation of cartilage samples with a mixed phenotype that combine characteristics of both hyaline and fibrous cartilage, as is the case with immature tissue in the early stages of chondrogenesis. Nevertheless, samples of cartilage tissue obtained using the studied protocols can be effectively used to model the processes of chondrogenesis in basic research. After optimization of the differentiation protocols, it is possible to use the resulting cartilage structures to make prototype cell products for preclinical and, possibly, subsequent clinical testing.