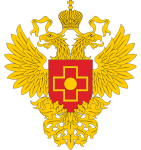
ORIGINAL RESEARCH
Molecular modeling and experimental confirmation of the search for agents mitigating toxic action of hydrogen sulfide
1 Apraksin Caspian Institute of Sea and River Transport — branch of the Volga State University of Water Transport of the Federal Agency for Sea and Inland Water Transport, Astrakhan, Russia
2 Astrakhan State Medical University, Astrakhan, Russia
3 Tatishchev Astrakhan State University, Astrakhan, Russia
Correspondence should be addressed: Ekaterina V. Trizno
Bakinskaya, 121, Astrakhan, 414000, Russia; ur.liam@0102-norien
Acknowledgments: the authors express their deepest gratitude to the departed Doctor of Chemistry, Professor N. M. Alykov and Doctor of Medical Sciences, Professor N. N. Trizno for their invaluable contribution to the development of scientific ideas and areas investigated by the authors of the article.
Author contribution: Golovatskaya LI — idea and design of the mathematical part of the study, analysis, study planning, data interpretation; Trizno EV — idea and design of the experimental part, coordination of the study, participation in the practical part of the experiment, manuscript authoring; Smirnova YuA — participation in the development of mathematical program, literature selection and analysis, discussion of the results; Trizno MN — practical arrangement of the experiment, data visualization, statistical analysis.
In the era of industrialization, hazardous emissions from plants and factories are a possibility. For example, at hydrogen sulfidecontaining (H2S) gas production and processing facilities, well equipment depressurization may lead to accidents involving combustion or spread of gas fluid without combustion. Today, it is necessary to be prepared for such accidents with gas. Among other things, violations of the production technology, like overspeeding during drilling of gas-saturated strata, make them more probable [1–6].
In such cases, hydrogen sulfide is a pathogenic factor that promotes disruption of the energy metabolism and boosts processes associated with the activity of free radicals in cells [7, 8]. The results of the said disruption and processes are damaged membranes and energy deficiency. Subsequently, on the one hand, the level of macroerg compounds goes down and content of Ca2+ ions in the cells goes up, and, on the other hand, the level of adenosine triphosphoric acid (ATP) decreases, which promotes shutdown of ion pumps and prevents ingress of Ca2+ ions from the intercellular medium, activation of membrane-binding phospholipases, hydrolysis of some phospholipids and reinforcement of permeability of the membranes [9, 10].
It can be assumed that development of various pathological conditions and diseases is associated with molecular changes in cell plasmalemmas. Being a target for H2S, membranes are involved in the pathological process that activates universal cell damage mechanisms associated with increased oxidation with free radicals and disruption of ion homeostasis [11–13].
In the recent years, active investigation of the molecular basis behind cell damage has boosted interest in the features of biological functioning of phospholipids [14, 15]. In biomembranes, lipid component is a functionally active matrix that integrates external influences and launches cellular control programs. There are three classes of membrane lipids: neutral lipids (30%), glycolipids (10%) and phospholipids (60%), the most widely represented class. The chemical energy of oxidized substrates or ATP is converted into electrical energy, namely, into a transmembrane difference in electrical potentials or into the energy of difference between concentration of substances contained in solutions separated by the membrane, and vice versa. Membranes can convert one form of energy into another. Retention of enzymes in mitochondria and oxidative phosphorylation, enzyme activity and cell sensitivity to hormonal and nervous regulation, as well as spatial identity depend on the state of the lipid component of the membrane [16–19].
The complexity of identification of the role of individual molecular mechanisms in realization of the processes that destroy membranes results from the closeness of their relationship. In this connection, deriving generalized descriptions of the cumulative patterns of cellular systems' reactions to pathological impulses of various origins not only promises better understanding of the general biological laws and how they function, but also allows re-evaluation of the methodology enabling adjustment thereof.
It is impossible to understand the actual behavior of a molecule without knowing its structure. Any change in the set of coordinates of the nuclei, as infinitesimal as it may be, builds a new geometry. The spatial structure of a molecule is not an inherent characteristic property of the system. Still, molecular structure allows pinpointing a certain temporary position of the molecule and grants understanding of the concept of its change. Mathematical modeling enables sequential investigation of various external factors. To determine the equilibrium spatial structure of the molecules, it is necessary to optimize the mutual arrangement of their atoms. Optimization of geometry means a search for atomic coordinates at which the system has the lowest energy value [20–22]. As a result of optimization, individual groups of atoms of a molecular system change their spatial position to a more favorable one in relation to neighboring atoms. Parametric method 3 (PM3) is one of the most consistent methods, carefully calibrated for a wide range of compounds, including organic and inorganic molecules of atoms of the main subgroups and hydrogenbonded systems. It conveys the structure, thermodynamics, dipole moments, ionization potentials, vibrational frequencies well. In case of simple organic compounds, it approaches the density functional theory (DFT) in accuracy but surpasses it in performance by dozens of times. The average error in determining the enthalpy of a formation is about 5 kcal/mol. The advantages of other semi-empirical methods over PM3 are mainly seen in evaluation of electronic, magnetic resonance parameters and electronic excitation spectra [21, 22].
From the chemical point of view, intermolecular electron transfer is a reduction/oxidation reaction, or RedOx. The processes of electron transfer in mitochondrial membranes form the physicochemical basis for the mechanisms of energy storing in a cell. Currently, it is assumed that the transfer of electrons between the cell's liquid phase components and intracellular proteins regulates the activity of cell proteins. Development of many diseases is associated with the increased levels of oxidants in the body; the list of such disorders includes atherosclerosis, liver cirrhosis, cataracts, arthritis, coronary heart disease, bronchial asthma, hepatitis, and diabetes. Albert Szent-Gy–rgyi, a Nobel Prize winner, wrote: "Balance between electron donors and acceptors with different biopotentials is one of the main parameters of life..." In the course of a redox reaction, the reduced form of one redox pair (reductant) donates electrons to the oxidized form (oxidizer) of another pair [23–25]. H2S is a known active ion exchange participant capable of binding copper atoms in cytochrome oxidase and thereby blocking the transfer of electrons from this mitochondrial respiratory chain enzyme to oxygen. Since body fluids are open systems constantly exchanging electrons with the environment, we discretized possible cross-reactions and used the liposomal membrane model in an aqueous medium.
The purpose of this work is to compare the degree of intermolecular interaction of two-component systems with a biomembrane (liposomal model thereof) in the reaction with hydrogen sulfide and acetylcysteine with the aim of adjusting the membrane's redox potential.
METHODS
Mathematical calculations were performed in GAMESS using the PM3 method (GBASIS=PM3), the gradient norm accuracy was up to the fourth decimal place inclusive (OPTTOL=1.0E-4). The results enabled building the z-matrix, the configuration of which is quite close to the point of global minimum of the potential energy surface. The calculation yielded the gradient value of 0.0000327 kcal/mol/angstrom, which makes the computational capabilities of GAMESS rather high compared to ChemOffice. The total energy value for the resulting configuration of molecules is calculated in atomic energy units and given in kJ/mol.
We use three criteria to increase the accuracy of the adsorption interactions model: the magnitude of charge transfer (Δq), the distance between atoms (Å) and thermal effects of the adsorption complexes (ΔEads).
Protein and phospholipid components were chosen as components of the cell membrane. The structure of the protein was a simple pentapeptide resulting from the calculation of the optimal equilibrium of the system from the viewpoint of the minimum total atomic energy. Given that phospholipid molecules can move from one side of the membrane to another, the in vitro model excludes variation in the biological response to pathogenic factors and minimizes the asymmetry of phospholipids in the membrane. As the base of liposomes, we used lecithin that was two-thirds phospholipids.
The liposomes were prepared as described below.
We prepared the sulfide solution within the first stage of hydrolysis as per the following molecular equation:
Na2S + HOH <=> NaHS + NaOH,
and the complete ionic equation for this reaction was as follows:
2Na+ + S2– + HOH <=> Na+ + HS– + Na+ + OH–.
We stopped the reactions before the second stage of hydrolysis since that would imply formation of volatile forms of hydrogen sulfide, which is toxic. Another reason behind this decision is the specific dosage approach adopted for this experiment.
We used a weighing unit (Conzept; Italy) with a weighing accuracy of 0.1 mg, a redox potential meter (in millivolts, mV) (Russia), a magnetic stirrer, chemical beakers and a cylinder (graduated, with a volume of 100 ml), pipettes, automatic dispensers, an ultrasonic disperser (China), powdered sodium sulfide, a fume hood.
The prepared solution of sodium sulfide (10%) had the sulfide concentration of 88 mg/l. Twelve grams of Na2S were mixed with 80 ml of distilled water (introduced thereto). We determined the exposure factoring in achievement of a stable change of the parameter at a constant level. The measurements were taken thrice, with subsequent derivation of the average values.
The liposome solution was prepared from soy lecithin (Protein company; Russia) based on isopropanol at the ratio of 30:70. Having added a 0.1% solution of methylene blue (for better visualization), we treated the solution with ultrasonic (US) waves for 10 minutes, and then added the liposome solution to the sulfide solution at the ratio of 1:1. The saturated solution of lecithin (1 g + 25 ml of isopropanol) was supplemented with 10 ml of ACC solution (10 mg/ml) and then exposed to ultrasonic waves. The redox potential was measured in solutions of liposomes with lecithin and ACC before and after immersion in a sulfide medium 15 minutes after preparation of the media.
Abnormalities of distribution were detected with the help of the Kolmogorov–Smirnov and Shapiro–Wilk tests. Numerical values are given as median and P5-, P95-percentiles. The differences were considered significant at p ≤ 0.05. For the analysis, we used descriptive statistical methods of Statistica 11.0 (StatSoft; USA).
RESULTS
Modeling the hydrogen sulfide's effect on a biological membrane includes generation of a mathematical model and a visual structure of the elements of the membrane and the toxicant. The plan implies a search for the sites of sorbate molecule that play a part in interactions resulting in the release of the greatest amount of energy. The energy of the activated complex should be at the minimum level possible. With the given structures, the interactions occur mainly in the amino group (NH2), sulfide (SH), carbonyl (CO), hydroxyl (OH), and methyl (CH3) groups, and may also involve hydrogen atoms of the benzene ring. Hydrogen sulfide can be bound both with hydrogen in amino and sulfide groups and with sulfur in amino, sulfide and hydroxyl groups. The strongest bonds include a hydrogen atom of the protein's SH group.
The tables below present the results of calculations of energy and geometric properties of the most significant molecular adsorption complexes (AC) with pentapeptide (tab. 1) and lecithin (tab. 2). The investigated amino acid sequence in the protein peptide was as follows: cysteine–phenylalanine– alanine–cysteine–tyrosine.
The tables show active sites of hydrogen bond formation under the influence of ACC and hydrogen sulfide on the components of the cell membrane. Transfer of the charge confirms that the interaction takes place, and the magnitude of the charge transfered indicates the direction in which the electrons move between the atoms of the interacting substances. The sign of the charge reflects the molecule acting on the membrane (H2S or ACC). Positive sign means the charge is redistributed from hydrogen in the substance to the more electronegative atoms of the membrane (fig. 1). Negative sign indicates the electrons move from the membrane's hydrogen to the "implanted" substances. The strongest interactions are observed between the lecithin's H35...S7 and ACC, in contrast to the hydrogen bonds of protein molecules and ACC (fig. 2). Participation of proton of the ACC's SH group in formation of the hydrogen bond translates into greater density of electrons of the sulfur atom, which boosts its nucleophilic properties. Active SH group in the molecule of ACC conditions the properties of this substance. The length of the formed bond is most typical for this type of interaction, and the adsorption energy value reflects the robustness of the adsorption complexes. In this case, lecithin is the preferred target (fig. 3).
Any change of interatomic positions affects the molecule's geometry as a whole, and hence alters the properties of the system, but a structured calculation allows registering the molecule's position in time and helps understand the principle of changes as they are shaped by external factors. As a result, the following tasks become more simple: establishing individual geometric positions of the molecules relative to each other; learning the peculiarities of the effect produced by the attacking substance; mapping active atoms of the membrane components; analyzing and showing the competitive replaceability of the antidote.
As shown, interactions of hydrogen sulfide and ACC have a lower minimum of the adsorption energy when reacting with lecithin than with peptide. This means we characterize such interactions as structures with more stable positions from the energy viewpoint. Therefore, the in vitro model is the lipid component of the membrane, and the liposomes formed have the diameter of 36.92 [27.98; 54.39] µm (fig. 4).
The redox potential of the sulfide solution was –718 [699; 723] mV. This is an extremely low value, which indicates the strength of reduction capabilities of the hydrogen sulfide ions. The redox potential of the saturated lecithin solution was –77 [–72; –81] mV. After ultrasonic treatment and preparation of the liposome solution with ACC, the redox potential was –54 [–41; –59] mV. The redox potential of the final solution of sulfides + lecithin + ACC has grown to –122 [–120; –131] mV.
Thus, introduction of liposomes with ACC (acting as an oxidizer) restored the redox potential. Consequently, when interacting with hydrogen sulfide ions, active SH groups of the ACC give the solution properties of a buffer.
DISCUSSION
Currently, researchers rely on quantum chemical calculations when studying models of systems of molecular complexes. Their works include models of complexes consisting of two or more molecules that were described (with underlying tasks executed manually) earlier. One of such works presented a model of interaction in a three component system of oil, water and demulsifier [26]. The inclusion of water as one of the molecular system's components can only be justified if the purpose is to investigate the details of the demulsification process in a specific case of a possibility randomly set by the user. An alternative approach is accounting for solvation [27], a feature available in any quantum chemistry program, like GAMESS. The research efforts in this field target specific problems and mainly focus on determining the 3D structure of the molecules, distribution of charge and electron density of each atom of a molecule, total energy of the molecules, energies and heat accompanying formation of molecules, energies of electrons, energies of nuclei and dipole moments of atoms that make up the molecule [28, 29].
This study focuses on a systematic consideration of the possible adsorption complexes emerging as the molecules form a hydrogen bond between them. The focus enabled sequential investigation of the process of immersion of membrane's components in a hydrogen sulfide environment with subsequent evaluation of introduction of ACC as a competitor for hydrogen sulfide. With the help of quantum chemical modeling, we established the key values of energy peculiar to the formation of reacting molecules and their systems. To establish the stable positions of adsorption complexes we have additionally determined and calculated three criteria for each of them: the adsorption energy, the charge transfered from one molecule to another and the size of the formed hydrogen bond. Having eliminated the structures that did not meet the criteria, we learned the signatures of active atoms on the molecular surfaces specific to this kind of interactions [21].
We prepared the liposomes using generally recognized methods [16–18]. Isopropyl alcohol was used as a solvent, as it is less toxic (3600 – 5740 mg/kg) compared to other organic solvents (xylene, chloroform) [30] and more economically sound compared to, for example, ethyl alcohol. We used a flow of gaseous nitrogen instead of a rotary alcohol evaporator, which greatly simplified the experiment.
CONCLUSIONS
In general, the suggested in silico method of mathematical modeling of action of toxic blockers on a cell membrane model can complement in vitro experiments. The results of this study allow a detailed description of the mechanism of events occurring on the cell membrane's surface. Further research will help improve understanding of the structure and properties of potential antidotes for a number of cytotoxic substances. Our data indicate that reaction sites for this type of interactions may form, and they predominantly appear on the atoms represented in the interaction signatures (fig. 1–fig. 3). The studied interaction between molecular components of biomembranes — protein and phospholipid, on the one hand, and hydrogen sulfide and ACC molecules "implanted" onto them, on the other hand, — is a good starting point for further investigations of the processes occurring on the surface of the cell membrane. Thus, it can be stated that lecithin is the most preferable target for an experimental study of the membrane component. Calculations of the energies of interaction of adsorption complexes and the redox potential of the systems show that lecithin combines more optimally with ACC compared to a combination with hydrogen sulfide. The oxidative environment created through addition of liposomes with ACC to a sulfide solution indicates that, from the energy viewpoint, the interaction of lecithin with ACC is better than with sulfide ions. Competitive hydrogen bonds between phospholipid and ACC with hydrogen sulfide in the background support further experiments. For example, a model of liposomes in a plasma solution will yield a better understanding and grounds for subsequent investigation of the interaction between molecules of ACC and hydrogen sulfide at a more complex organ level of biosystem organization.