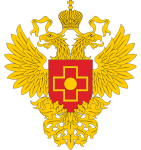
ORIGINAL RESEARCH
The relationship between the variants of immune response and the cortisol and adrenaline levels associated with cooling
N. Laverov Federal Center for Integrated Arctic Research, Ural Branch of the Russian Academy of Sciences, Arkhangelsk, Russia
Correspondence should be addressed: Veronika P. Patrakeeva
Nikolsky prospect, 20, Arkhangelsk, 163020, Russia; ur.xednay@akinorev.aweekartap
Funding: the study was performed as part of the Program of Fundamental Scientific Research on the topic of the environmental immunology laboratory, Institute of Physiology of Natural Adaptations, N. Laverov Federal Center for Integrated Arctic Research, Ural Branch of the Russian Academy of Sciences (project № 122011300377-5).
Author contribution: Patrakeeva VP — study planning, data acquisition, processing and interpretation, literature review, manuscript writing; Kontievskaya EV — data acquisition and processing, literature review.
Compliance with ethical standards: the study was approved by the Ethics Committee of the N. Laverov Federal Center for Integrated Arctic Research, the Ural branch of RAS (protocol № 4 of 7 December 2016, protocol № 6 of 14 February 2022) and conducted in accordance with the principles of the Declaration of Helsinki (1975, rev. 2013).
Interaction between the immune and nervous systems ensures the development of adaptive responses to living conditions. There are neurotransmitter receptors on the membranes of immunocompetent cells, through which neurotransmitters can affect the cells’ functional and metabolic activity.
However, T cells can control both synthesis and degradation of neurotransmitters [1, 2]. The cold exposure leads to the changes in the levels of hormones involved in instant and longterm adaptation. The development of adaptive physiological processes involves mainly two systems: the sympathetic– adrenal–medullary and hypothalamic-pituitary-adrenal axes. Low blood levels of adrenaline, the hormone of the sympathetic– adrenal–medullary axis, are reported in the normal state, while the exposure to stressor results in the significant increase in adrenaline concentration that intensifies catabolic processes. Catecholamines control a number of leukocyte functions, such as proliferation and differentiation, mitogenic responses, lytic activity of natural killers and cytokine production. High doses of catecholamines cause abnormal platelet aggregation [3–6]. Cortisol, the steroid hormone of the hypothalamic-pituitaryadrenal axis, can elevate blood glucose levels and suppress the immune system, thereby inducing apoptosis in proinflammatory T cells, it also can suppress antibody production by B cells and reduce migration of neutrophils during inflammation [7]. Cortisol reduces glycogen synthesis and contributes to the fat, protein and carbohydrate metabolism via gluconeogenesis [8]. Gluconeogenesis makes it possible to respond quickly to the changes in the need for ATP. Intensification of lipolysis in the adipose tissues under exposure to cortisol facilitates the release of glycerol and free fatty acids that are also involved in β-oxidation and are used as a source of energy by other cells. The effects of cortisol exposure result mostly from the duration of exposure and the dose. Activation of catabolic mechanisms aimed at meeting the body's energy needs is justified in case of short-term stress, while chronic exposure to the stressor and the long-term immunosuppressive effects of high cortisol doses can result in the body’s adaptation reserve depletion. Even people residing permanently in the North show different degrees of adaptation to specific environmental conditions, including cold exposure. This is largely due to baseline levels of the neuro-immuno-endocrine system activation and the spare capacity enabling adequate responses to external stimuli. The study was aimed to determine the relationship between the baseline levels of cortisol and adrenaline, as well as the changes in their concentrations associated with the adaptive immune response to whole body cooling.
METHODS
The changes in immunological, hematological and biochemical parameters of peripheral venous and capillary blood were studied in 173 generally healthy people. Inclusion criteria: no acute disorders at the time of examination; non-use of cold exposure training; age 20–60 years. Blood was collected twice by skilled medical specialists: 1) before staying in the USHZ-25N cooling chamber (Xiron-Kholod; Russia) at –25 °С for 5 min and 2) immediately after staying in the chamber. Blood serum and plasma were separated by centrifugation, the samples were freezed once at a temperature of –20 °С. Assessment was performed in the morning (8 to 10 am), strictly in the fasting state. Differential blood count was determined using the XS-1000i hematological analyzer (Sysmex; Japan). Enzyme immunoassay was used to determine the levels of adrenaline with the test kit (IBL, Hamburg; Germany), as well as the levels of cortisol (DBC; Canada) and ferritin (ORGENTEC Diagnostika GmbH; Germany). The results were assessed using the Multiskan FC microplate photometer (Thermo Scientific; Finland). The levels of glycogen in lymphocytes were determined by the cytochemical method (Abris+; Russia) involving calculating the percentage of positively stained cells with the Biomed 4 LED microscope (Biomed; Russia). The study results were processed with the Statistica 6.0 software package (StatSoft; USA). The data were described using the median (Ме) and 25–75th percentiles. The nonparametric Mann–Whitney U test was used to determine the significance of differences. When testing the statistical hypotheses, the significance level (p) was considered to be 0.05.
RESULTS
We compared the data in the groups depending on the changes in peripheral blood lymphocyte counts after the shortterm whole body cooling. In group 1 (n = 52) the lymphocyte counts decreased by 1.5–2 times (from 2.1 (1.77; 2.44) × 109 to 1.69 (0.95; 2.16) × 109 c/L (p < 0.001)), in group 2 (n = 42) these increased from 1.49 (1.26; 1.74) × 109 to 2.22 (1.48; 2.61) × 109 c/L (p < 0.01), in group 3 (n = 79) no significant differences were revealed (1.88 (1.46; 2.17) × 109 and 1.82 (1.46; 2.56) × 109 c/L). The development of adaptive response associated with the decrease in the circulating lymphocyte counts (group 1) took place against the background of relatively high cortisol levels (317.4 (283.5; 732.2) mmol/L), the levels of which increased to reach the upper limit of normal range, i.e. 606.3 (281.0; 963.2) mmol/L, after the whole body cooling in this group. When peripheral blood lymphocyte counts increased in response to whole body cooling (group 2) or no significant differences were revealed (group 3), the baseline cortisol concentration was actually 2 times lower and did not change after the cold exposure (fig. 1).
Glucocorticoids that are the main effector molecules of the hypothalamic-pituitary-adrenal axis ensure mobilization of energy substrates into the bloodstream. Cortisol can alter bioenergetic cell fuctions via transactivation or transrepression of target nuclear and mitochondrial genes, as well as activation of cytoplasmic signaling pathways, thereby affecting mitochondrial activity. ATP production largely depends on the cellular oxygen consumption via the oxidative phosphorylation system (OXPHOS) located in the inner mitochondrial membrane [9–11]. It is well known that the short-term exposure to cortisol induces mitochondrial biogenesis and enzyme activity of some OXPHOS subunits, causing higher mitochondrial activity, since the long-term exposure to high doses of cortisol results in the decrease in mitochondrial activity, OXPHOS dysfunction, increased production of reactive oxygen species, and structural abnormalities [12]. Thus, higher baseline cortisol levels and their further increase in response to cold exposure can be associated with the decrease in functional activity of mitochondria observed in case of glycolytic activity intensification. On the one hand, intensification of glycolysis is necessary for reprogramming of lymphocytes, their rapid activation. On the other hand, according to the model of mitochondrial allostatic load, mitochondrial function can determine the limits of the human ability to adapt to external stressors, whereby the higher mitochondrial content and function are associated with the increased adaptability and biological stability potential, while the decreased mitochondrial function limits the ability to adapt and opens the door to stress-related disorders [13]. We have found that the adaptive response to cooling, accompanied by the decrease in circulating lymphocyte counts against the background of long-term exposure to higher cortisol concentrations, is associated with intensification of glycolysis and the decrease in the glycogen content of lymphocytes from 4.01 to 2.83% (p < 0.01), which is essential for effective ATP production in case of immunocompetent cell activation.
Adrenaline is an effector mediator of the sympathetic– adrenal–medullary axis. There no differences in the baseline adrenaline levels between three groups: in group 1 the level was 30.7 (25.7; 43.8) ng/mL, in group 2 it was 30.0 (29.14; 44.6) ng/mL, and in group 3 it was 32.5 (26.38; 39.0) ng/mL. The significant increase in adrenaline concentrations was revealed after the whole body cooling in the subjects, for whom the increased lymphocyte counts were reported (group 2) (fig. 2). No significant changes in the concentrations of this catecholamine were revealed in groups 1 and 3.
Adrenaline causes the increase in succinate dehydrogenase activity and inhibits α-ketoglutarate dehydrogenase activity in mitochondria of peripheral blood lymphocytes [14], reduces oxidative phosphorylation in mitochondria, and causes mitochondrial dysfunction [15, 16]. The elevated adrenaline levels found in group 2 are associated with no changes in the glycogen content of lymphocytes (3.6% before cooling, 4.8% after cooling, respectively). This is probably due to the adrenaline ability to increase the ADP phosphorylation time by more than two times, thereby significantly reducing the ATP synthesis rate.
The stress-induced alterations due to adrenaline and cortisol exposure can affect the iron metabolism. The levels of ferritin are considered as an indirect criterion of adequate iron levels. On the one hand, these represent the iron depot, and on the other hand these are considered as a marker of acute inflammation, while the increase in blood ferritin levels can be associated with inflammatory tissue destruction. Iron ensures effective adaptation to cold, maintains energy balance and thermogenesis [17]. Assessment of the correlations of the adrenaline, cortisol and ferritin levels associated with various types of response to whole body cooling has shown that baseline ferritin levels in groups 1 and 2 are almost the same, while in group 3 lower ferritin levels are reported that significantly increase after the cold exposure while still within normal range (fig. 3).
DISCUSSION
Adaptation to low temperatures is ensured by interaction between the immune and endocrine systems. Exposure to external factors affects the body in different ways depending on its baseline state and the ability to respond, i.e. the reserve capacity to form instant and long-term adaptive responses. The exposure to stressor results in the adrenal gland activation, secretion of adrenaline and cortisol capable of changing the number of immunocompetent cells in the bloodstream.
However, the research data are controversial. There are data supporting both stimulation and immunosuppressive effects, and the mechanism of such interaction is poorly understood [18–21]. Furthermore, enough iron stored is a factor that is important for realization of adaptive responses to hypothermia. Ferritin, that represents the iron depot and regulates the iron metabolism, controls the integrity and function of mitochondria in the cells, thereby ensuring the energy and thermal homeostasis [22]. Our findings show that the increase in the baseline activity of the hypothalamic–pituitary–adrenal axis in response to the whole body cooling results in intensification of glycolysis and the decrease in the circulating lymphocyte counts that may be due to migration of these. At the same time, prolonged exposure to high concentrations of cortisol can be considered as the chronic stress that has an effect similar to that of the long-term antigenic stimulation and can contribute to production of lymphocytes showing signs of replicative aging [23]. The increase in the circulating lymphocyte counts after the cold exposure associated with the sympathetic–adrenal– medullary axis activation can be mediated by the enchanced release of cells from the depot or the change in the ratio of the marginated and circulating lymphocyte pools caused by vasoconstriction. No changes in lymphocyte counts in individuals subjected to whole body cooling are associated with no significant changes in the concentrations of cortisol and adrenaline, but with significant changes in ferritin levels. Cooling contributes to the ferritin heavy chain degradation, thereby causing ferroptosis, release of free iron ions, induction of reactive oxygen species accumulation, and inhibition of the glutathione- glutathione peroxidase 4 (GPX4) pathway [24, 25].
CONCLUSIONS
It has been found that the variant of response to whole body cooling is correlated mainly with activation of the sympathetic– adrenal or hypothalamic-pituitary-adrenal axis. Low baseline levels of cortisol and adrenaline along with no changes in their concentrations after the whole body cooling are not associated with intensification of glycolysis in lymphocytes. On the one hand, this suggests more stable condition of the body and no stress caused by such cold exposure. On the other hand, elevated ferritin levels can be indicative of inflammation. The effects of cortisol and adrenaline are associated with the changes in the circulating cell counts and metabolic activity of these cells. The higher baseline levels of cortisol cause intensification of glycolysis together with the decrease in glycogen content and therefore further intense ATP production that is essential for activation of immunocompetent cells. In this context the decrease in circulating lymphocyte counts is observed that may be due to rearrangement of functionally active cells in the tissue aimed at providing the effective immune defense. Possibly due to decelerating the rate of ADP phosphorylation, adrenaline does not allow such a rapid (within 5 min) increase in glycolytic activity of lymphocytes. The increase in adrenaline concentration in response to the cold exposure is associated with elevation of circulating lymphocyte counts, since the duration of exposure eliminates the possibility of lymphopoiesis. This fact can be explained by rearrangement of cells from the marginated to the circulating layer due to vasoconstrictor effects of adrenaline or the release of cells from the depot. The data obtained add to the knowledge about the role of the neuro-immuno-endocrine regulation in the development of individual cold sensitivity.