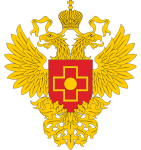
This article is an open access article distributed under the terms and conditions of the Creative Commons Attribution license (CC BY).
ORIGINAL RESEARCH
Therapeutic efficacy of N-cholinolitic drug N,N-Diethyl-5,5-diphenyl-2-pentynylamine in models of non-cardiogenic pulmonary edema
Golikov Research Clinical Center of Toxicology of the Federal Medical and Biological Agency, Saint-Petersburg, Russia
Correspondence should be addressed: Konstantin A. Krasnov
Bekhtereva, 1/2, k. 54, Saint-Petersburg, 199106, Russia; ur.liam@xot_vonsark
Funding: state contract № 2124388100122000000000000/64.020.21.9 of 2 November 2021, “Developing New Antidote Medications for Treatment of Toxic Pulmonary Edema” (code: “Tuman”)
Author contribution: Melikhova MV — assessment of the animals’ physiological state, data processing and interpretation; Krasnov КА — modeling of toxic pulmonary edema in animals, prepress manuscript preparation; Rozhko МА — drug administration through inhalation and monitoring of external respiration parameters in animals; Lapina NV — general management; Krasnova АА — lung gravimetry in animals.
Compliance with ethical standards: all the procedures involving model animals were performed in accordance with the principles of Good Laboratory Practice and the Directive 2010/63/EU of the European Parliament and (2010) on the protection of animals used for scientific purposes.
Non-cardiogenic pulmonary edema (NCPE) is a life-threatening condition defined primarily as acute lung injury syndrome and its most severe form, acute respiratory distress syndrome [1], as well as severe bilateral pneumonia. These conditions are characterized by rapid progression of hypoxemia and hypoxia resistant to oxygen therapy [2, 3]. NCPE may result from poisoning with pulmonotoxic chemicals (often associated with fires, chemical accidents and other extreme situations) or from viral or bacterial pneumonia [3, 4], septic or hemorrhagic shock, multisystem organ failure, and other causes [5]. The mechanisms underlying these processes are different: toxic pulmonary edema (TPE) results from primary blood–air barrier damage [4], and cardiogenic edema results from the blood pressure increase in pulmonary circulation by more than 30 mmHg.
Pulmonotoxic effects are exerted by the substances capable of damaging alveolar membranes of the lung, such as phosgene, chlorine, dimethyl sulfate, nitrogen oxides and sulfur oxides, ammonia, acids, thiourea, and other volatile toxic agents [6, 7]. Inhalation of vapors or aerosols of some toxic agents (dichloroethane, trichloroethylene, etc.) results in mixed pulmonary edema showing similarities to both toxic and cardiogenic pulmonary edema [8]. The common mechanisms of the blood–air barrier damage also underlie the development of pulmonary edema caused by viral and bacterial infections [5].
The main focus of the ARDS therapy is to ensure adequate gas exchange by selecting the respiratory support conditions [9]. NCPE therapy involves the use of hyperbaric oxygen therapy [10] and other respiratory support methods [9, 11], as well as some categories of drugs: ganglionic blockers (such as pentamine), narcotic analgesics (morphine), cardiac glycosides (strophanthin, digoxin), diuretics (lasix), corticosteroids (prednisolone), antifoaming agents [12, 13], antihypoxants [14]. Sedatives, such as ketamine, central α-2 agonists, benzodiazepines, etc., have been proposed as pharmacological agents for treatment of TPE [15].
The approaches to TPE treatment continue to be developed. Thus, recently the aquaporin-5 blockers [16], innovation technologies involving the use of perfluorocarbon liquids [17], recombinant heat shock proteins (including those combined with pulmonary surfactant) attract attention in this context [18, 19].
However, no uniquely effective agents and methods for prevention and treatment of TPE caused by pulmonotoxic chemicals have been developed so far [20, 9]. Mortality among affected individuals with TPE reaches 60% even in hospital settings, and the vast majority of this number die within three days after the exposure to toxic chemical [5, 6].
Thus, the development of new, more effective anti-edematous drugs is a pressing issue of modern medicine.
Among potential remedies for treatment of NCPE, N,N-diethyl-5,5-diphenyl-2-pentynylamine hydrochloride (DDPA) synthesized in the Golikov Research Clinical Center of Toxicology of FMBA of Russia, is of interest. Pharmaceutical substance has been registered and is produced by the Federal State Unitary Enterprise Research and Production Center "Farmzashita" of FMBA of Russia (№ Р N002888/01 of 6 June 2008). The structural formula of the active ingredient is provided in figure.
Pharmaco-toxicological assessment of DDPA has been reported earlier [21]. DDPA is an active N-cholinolitic agent, it shows pronounced antispasmodic and anti-inflammatory activity, local anesthetic effect, improves blood circulation, and exerts antioxidant and membrane stabilizing effects. According to the available data [21], DDPA has a beneficial effect on the inhalation injury caused by pulmonotoxic chemicals and irritants, allowing one to expect its efficacy against TPE. This is also suggested by the previously published data [22] showing the DDPA therapeutic effect in the model of non-cardiogenic pulmonary edema.
The study was aimed to determine the therapeutic efficacy of DDPA in the animal models of toxic pulmonary edema.
METHODS
The N,N-diethyl-5,5-diphenyl-2-pentynylamine hydrochloride (DDPA) pharmaceutical substance was provided by the Federal State Unitary Enterprise Research and Production Center "Farmzashita" of FMBA of Russia (99.7% purity). The study involved the use of the “chemically pure” and “pure for analysis” reagents.
The experiments involved male white outbred rats (age three months, body weight 200–220 g) obtained from the Rappolovo animal nursery (Leningrad region, Rappolovo, Russia; veterinary certificate № 15806716021). Animals were kept under standard conditions with the 12 h light/dark cycle and free access to water and food. Rats were selected for the experiment by randomization.
Pulmonary edema modeling and experimental design
Two methods were used to create the rat models of acute NCPE. In the first case, pulmonary edema was induced by intraperitoneal thiourea injection, and in the second case it was induced by the nitrogen dioxide inhalation [5].
Method to induce NCPE through intraperitoneal thiourea injection
The rats received intraperitoneal injections of the 10% thiourea aqueous solution in a dose of 100 mg/kg. Immediately after that the animals were put in the 100 L inhalation chamber and kept in the atmosphere containing carbon monoxide at a concentration of 1500 mg/m3 for 30 min to enhance pulmonary hypoxia. The concentration of СО in the chamber was monitored using the automatic gas analyzer. The experiment involved 80 male outbred rats divided into two groups: the control group (no treatment) and the group treated with DDPA. An hour later 20 animals were randomly selected in each group; macroscopic features of the lungs, pulmonary coefficient (PC), lung dry weight (LDW) measured as a percentage, lung hydration status (LHS) measured as a percentage were assessed in these animals. The rest of 40 animals were used to estimate survival rate. The results are provided in tab. 1.
Method to induce NCPE through NO2 inhalation
During the experiment cages with animals were put in the 100 L inhalation chamber, nitrogen dioxide was supplied from the cylinder with the air-gas mixture. A total of 10 experimental rats were distributed into four cages, 2–3 animals per cage. NO2 concentration in the chamber was monitored using the MONOLIT gas analyzer (Nord-West Engineering; Russia). At the same time, the concentrations of СО2 (with the PKU-4/1-МК-S gas analyzer, Russia) and О2 (with the PКG-4-К-N-1-М gas analyzer, Russia) in the chamber were monitored and maintained within normal limits. The rats were kept in the inhalation chamber for 18 min after the specified NO2 concentration was established, the total inhalation time was 20 min. NO2 concentration fluctuations in the chamber did not exceed 5% of the specified value.
Preliminary tests were used to determine the nitrogen dioxide toxic concentrations: LC20/20min = 180 mg/m3, LC50/20min = 210 mg/m3, and LC80/20min = 225 mg/m3. These experiments involved 40 animals.
After selection of toxic concentrations, the experiments focused on assessing the DDPA therapeutic effects in TPE were conducted. In the first experiment involving 60 animals, we assessed the effects of DDPA on the survival rate of rats exposed to three nitrogen dioxide concentrations (tab. 2). The second experiment involving 72 animals was focused on assessing the external respiration parameters and gravimetric pulmonary coefficients in survivors after exposure to the nitrogen dioxide concentration LC50/20min = 210 mg/m3 (tab. 3, tab. 4).
Drug administration and assessment of treatment outcome
The animals were divided into two groups immediately after the end of the NCPE induction procedure. Animals of the first group were administered 1% aqueous solution of DDPA via inhalation, and animals of the second group (controls) were administered pure water via the same route. The DDPA solution inhalation time was 2 min. The second DDPA solution inhalation was applied an hour later. The experiments involved the use of the OMRON NE-C28 PLUS nebulizer (China) with the nebulizing rate of 0.5 mL/min. The average generated aerosol particles dispersion was 1.5 µm, and the aerosol particle size distribution ranged between 0.2 and 10 µm.
The treatment outcomes were assessed relative to the control group. The animals’ survival rate, overall health condition, clinical manifestations of poisoning, macroscopic features of lung injury, and gravimetric pulmonary coefficients were assessed in accordance with the common diagnostic criteria of pulmonary edema in laboratory animals [23]. The rats’ external respiration parameters were measured using the М150Р system (BIOPAC Systems; USA): respiratory rate per minute (RR); inspiratory capacity in mL (IC). The external respiration parameters and lung gravimetry were assessed before the exposure (baseline), 2 and 5 h after the exposure. Gravimetry was performed only in survivors withdrawn from the experiment by decapitation in groups of 12 animals per group.
The following gravimetry parameters were used to assess the NCPE severity: pulmonary coefficient (PC), lung dry weight (LDW) and lung hydration status (LHS) calculated according to formulas 1–3.
m1 — weight of the experimental animal’s fresh lungs (g);
m2 — body weight of the experimental animal (g).
m1 — weight of the experimental animal’s fresh lungs (g);
m3 — weight of the experimental animal’s dried lungs (g).
m1 — weight of the experimental animal’s fresh lungs (g);
m4 — weight of the intact animals’ fresh lungs (g).
The weight of the dried lungs (m3) was determined by drying the animal’s fresh lungs in the drying chamber at 100 °С until the constant weight was achieved.
Statistical data processing
Statistical processing of the results performed using descriptive statistics involved calculation of the mean and standard error of the mean determined based on the standard deviation. The data obtained were tested for normality using the Shapiro–Wilk test. When proving the normal distribution of data in the experimental groups and the equality of variance, the parametric Student's t-test implemented in the StatPro application was used. The differences were considered significant at p < 0.05 [24, 25].
RESULTS
Table 1 (tab. 1) shows the treatment outcomes of NCPE induced by intraperitoneal thiourea injection in rats.
After inducing NCPE, the animals were put in the cage where they were kept to follow-up. Hypodynamia and dyspnea were observed in all animals 40–60 min after administration of thiourea. Later the control animals’ condition continued to deteriorate progressively: accessory muscle breathing, adynamia, cyanosis, and disheveled fur showed up. Eventually, some animals of the control group died; death occurred within 1.5–2.0 h in conditions of the acute respiratory failure progression. After that no animals died during the follow-up period.
The condition of animals, treated with 1% DDPA solution immediately after the inhalation, stabilized, and no respiratory system-related worsening occurred. Motor activity and grooming were reported in rats.
Assessment of macroscopic features of the lungs in the animals that were withdrawn from the experiment revealed enlarged lungs, and burgundy color liquid discharge was observed in the section of parenchyma. The dark-red exudate in the amount of up to 5 mL was detected in the thoracic cavity. In the group of animals treated with DDPA, pale-yellow pleural effusion with no hemorrhagic component was observed.
The results obtained when studying the efficacy of nitrogen dioxide-induced NCPE treatment are provided in tab. 2–tab. 4.
Table 4 presents gravimetric pulmonary coefficients reported in animals at different times (2 h and 5 h) after poisoning compared to that of intact animals.
Clinical manifestations of TPE in animals after exposure to nitrogen dioxide
Hypodynamia and dyspnea were observed in animals after the exposure in the chamber containing NO2. After that the animals’ condition worsened: accessory muscle breathing, adynamia, cyanosis, and disheveled fur showed up. The animals died as a result of progressive acute respiratory failure. Autopsy showed that the dead animals’ lungs were filled with foamy fluid. Animals died in both groups, subjected and not subjected to treatment, however, the condition of animals that received DDPA inhalations stabilized, in contrast to the control group.
DISCUSSION
The treatment outcomes of NCPE caused by intraperitoneal thiourea administration that are provided in tab. 1 indicate the decrease in the pulmonary edema severity. No fatal cases along with significant decrease in gravimetric pulmonary coefficients are observed in the group of animals receiving DDPA inhalations. Assessment of pulmonary weight indices in animals shows that administration of the drug slows down the buildup of fluid in the lungs [24], thereby reducing the pulmonary edema severity.
According to the treatment outcomes of NCPE caused by NO2 inhalation (tab. 2), treatment with DDPA reduces mortality rate relative to controls. The DDPA beneficial effects were more obvious in animals intoxicated with medium concentration of LC50/20min = 210 mg/m3 within the period of 2–5 h after exposure. Furthermore, DDPA increased the rats’ life expectancy: significant differences from controls were revealed in animals intoxicated with low and medium NO2 concentrations (LC50/20min = 210 mg/m3). Treatment with DDPA results in the decrease in respiratory rate (RR) and the increase in inspiratory capacity (IC) relative to the control group within 5 h. Gravimetric pulmonary coefficients (tab. 4) suggest inhibition of the buildup of fluid in the lungs of animals receiving DDPA. Significant differences from controls have been obtained for pulmonary coefficient (PC), other parameters demonstrate a trend of declining lung hydration status.
Thus, the findings demonstrate the efficacy of DDPA therapy in the TPE models used. The issue of the mechanism underlying realization of the DDPA therapeutic effect remains open. As the available data on the DDPA pharmacology might suggest, its effect is probably of combined nature. Due to its antispasmodic activity, DDPA can reduce blood pressure in pulmonary circulation. Presumably, this in combination with membrane stabilizing and antioxidant activity reduces lung hydration.
It should be noted that the treatment outcomes of TPE induced by intraperitoneal administration of thiourea are better than that of edema caused by NO2 inhalation. Such differences can be explained by the fact that nitrogen dioxide causes a more deep irreversible lung tissue injury than thiourea. Thiourea is rapidly excreted from the body, it does not form stable adducts, and its damaging effects start to wear off almost immediately after the decrease in its blood levels. In contrast, nitrogen dioxide reacts with lung tissues, producing toxic metabolites and inducing destructive processes throughout the long period after poisoning. That is why toxic pulmonary edema caused by NO2 is extremely difficult to treat.
CONCLUSIONS
The study has shown that inhalation of DDPA solution has a therapeutic effect in two models of toxic pulmonary edema. The efficiency of treatment with DDPA is higher in the model of TPE caused by intraperitoneal tiourea administration than in the model of NO2-induced TPE. Extrapolation of the data obtained to humans suggests that the use of DDPA in individuals with injuries induced by pulmonotoxic chemicals may be promising at the prehospital stage and during transfer to the hospital for treatment.