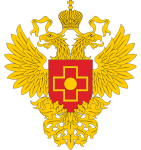
ORIGINAL RESEARCH
Effects of mild hypothermia on the cerebral microvascular tone
Pavlov Institute of Physiology, Russian Academy of Sciences, Saint Petersburg, Russia
Correspondence should be addressed: Nadezhda N. Melnikova
nab. Makarova, 6, Saint Petersburg, 199034; ur.liam@nnlem
Funding: the study was supported by the State Programme 47 GP “Scientific and Technological Development of the Russian Federation” (2019–2030), project 0134-2019-003.
Compliance with ethical standards: the study was approved by the Ethics Committee of the Commission for Care and Use of Laboratory Animals, Pavlov Institute of Physiology RAS (protocol № 05/10 of 10 May 2021) and conducted in accordance with the principles of the Declaration of Helsinki (2013).
Blood loss associated with trauma is a major factor of high mortality among both civilians and servicemen [1, 2]. In the majority of cases severe condition of the patient with hemorrhage is aggravated by accidental hypothermia [3–5].
According to the existing guidelines on treatment of traumatic bleeding, hypothermia should be avoided [6, 7]. However, in early 2000s the animal experiments were conducted as part of the number of foreign trials that involved simulation of hemorrhagic shock and the use of cooling. The majority of studies involving various animal species were focused on the effect of different temperatures on survival rate. Thus, in rats with lethal uncontrolled bleeding, cooling of the body surface to 34 and 30 °C resulted in the increased survival (119 and 132 min, respectively, vs. 51 min) compared to normothermic rats [8]. The same results were obtained for rats with controlled hemorrhagic shock cooled to 34 °C within 12 h and during resuscitation [9]. Therapeutic mild hypothermia (34 °C) and delayed fluid resuscitation improved survival of rats after uncontrolled hemorrhagic shock [10].
The swine models of hemorrhagic shock also showed improved survival associated with hypothermia. Thus, surface cooling to Тr 34 °C improves survival of pigs after prolonged controlled hemorrhagic shock and splenic rupture [11]. Deep hypothermia and cardiopulmonary bypass can improve survival in pigs with lethal uncontrolled hemorrhage [12]. The study of traumatic uncontrolled hemorrhagic shock in rabbits has shown that mild hypothermia (Тr 34 °C) after restoration of circulation improves early survival and the function of organs, not disturbing homeostasis [13].
Later attempts were made to explore neuroprotective mechanisms of hypothermia. One of the recent reviews provides evidence of the effectiveness of using therapeutic hypothermia during heart surgery, in traumatic brain injury and neonatal encephalopathy in both animal studies and clinical trials [14]. Prevention of irreversible neuronal necrosis and ischemic brain injury is the main result of using hypothermia.
The use of therapeutic hypothermia in clinical practice in cases of hemorrhagic shock and resuscitation may be effective in terms of reducing the levels of reactive oxygen species and decreasing vascular permeability [15], contributing to successful resuscitation of the patient. It has been proven that the use of therapeutic hypothermia (34 °C) in rats with controlled or uncontrolled hemorrhagic shock reduces blood loss and oxygen consumption and causes no coagulopathy [16]. The ovine model has shown that the use of mild hypothermia in healthy animals is associated with significantly reduced density of sublingual capillaries along with no pronounced changes in systemic hemodynamics [17].
Beneficial effects of mild hypothermia on the cerebral cortex microcirculation measured by multichannel laser flowmetry have been proven in the rabbit model of cardiac arrest [18]. A similar effect of cerebral microcirculation improvement was observed in rats subjected to early mild whole-body hypothermia during resuscitation from cardiac arrest [19]. At the same time, mild hypothermia reduced both cerebral blood flow in the pigs’ microvessels, as well as the cerebral metabolism after blood circulation restoration relative to normothermic animals [20, 21]. The overall effect was as follows: the ratio of the need and oxygen supply to the brain improved during the period of hypothermia.
A pilot study focused on assessing the effects of hypothermia on microcirculation in severe hemorrhagic shock was performed in 2021 [22]. The study showed that despite the fact that hemorrhage caused severe microcirculation disorder in the sublingual area, intestinal villi and the kidney cortex of the sheep, further decrease of rectal temperature to 33–34 °C improved microcirculation in these areas.
In our previous study, immersion cooling of the body aggravated microcirculatory dysfunction cause by acute massive blood loss [23]. When the rats were cooled so fast, additive effects of hypothermia and hemorrhage on vasoconstrictor responses of cerebral microvessels were observed in the phase of moderate hypothermia. To determine the pattern of mutual effects of hypothermia and hemorrhage, we set a goal to explore the effects of mild hypothermia in rats with previously evoked moderate blood loss on the brain microcirculatory bed.
METHODS
The experiments involved male Wistar rats (n = 23) with the body weight of 280–310 g obtained from the Collection of Laboratory Mammals of Different Taxons (Pavlov Institute of Physiology RAS).
The animals were anesthetized with urethane solution (intraperitoneal aministration, 1000 mg/kg). To perform an in vivo study of cerebral microvessels, parietal craniotomy with the bone flap size of 7 × 5 mm was performed, and dura mater was removed within the window. A catheter filled with heparinized (40 U/ml) saline was placed in the left femoral artery for direct blood pressure measurement, and the right femoral artery was catheterized for blood collection. The rectal temperature (Tr) of 37–38 °С was maintained in the rats during the surgical procedure using an electric pad for small animals.
The animals were randomized into three groups during the experiments. Animals of group 1 (n = 7) were cooled to the rectal temperature of 34 °С, then this temperature was maintained throughout 3.5 h of the experiment. Animals of group 2 (n = 6) were used to simulate blood loss with the estimated rate of 20% of total blood volume, then the animals with the baseline Тr 37 °С were kept at the room temperature of 20–22 °С throughout 3.5 h of the experiment. After the evoked hemorrhage, animals of group 3 (n = 10) were cooled in the air to Tr 34 °С that had been maintained for 3.5 h.
Arterial blood was collected: 1.2 mL per 100 g of the animal’s weight or 20% of total blood volume. This was equivalent to moderate blood loss, i.e. blood exfusion in the rat with the body weight of 300 g was 3.6 mL. Blood was collected for ≈10 min, the average exfusion rate was 0.36 mL/min.
Rats were cooled to Тr 34 °С in the air at standard room temperature by wetting a part of the animal’s back and using the directional airflow produced by a domestic fan. The cooling duration was ≈ 30 min.
The order of exposures used during the experiment in each group is provided in fig. 1.
Imaging and monitoring of the pial microcirculatory bed was performed using the vital microscopy system that included the LUMAM К-1 microscope (LOMO; Russia) with the contact dark-field lens and the ACUMEN AiP-B84A color video camera (ACUMEN Int. Corp.; Taiwan). The resulting image was subjected to computer processing in the Pinnacle Studio software package. The calibration measurement was performed using the OS-1 standard stage micrometer (scale division value 10 µm).
After preliminary procedures (cooling / exfusion / both) the responses of pial arteries were recorded every 15 min throughout 3.5 h of the experiment. The baseline microvessel diameter was 10–40 µm, and the majority of arteries were about 20–25 µm in diameter. Identical segments of blood vessels were measured 50–90 times within each series of the experiment at each time period. After the end of the experiment animals were euthanized via urethane solution overdose.
Heart rate according to ECG, average blood pressure (direct measurement via femoral artery catheter) and respiratory rate (carbon sensor) were continuously recorded. The Е-154 ADC (L-Card; Russia) was used to digitize analog signals and save these signals in computer memory.
Statistical processing of the results was performed using the STATISTICA 6.0 software package. Significance of intragroup differences was assessed using the nonparametric Wilcoxon test, while intergroup differences were revealed using the nonparametric Mann–Whitney U test. The significance level was set as р < 0.05. All experimental data were presented as mean ± standard error of the mean (M ± SE).
RESULTS
Indicators of the body’s functional state before exposure (referred to as normal) were the same (р ˃ 0.05) in all experimental groups (table). In groups 2 and 3, preliminary blood exfusion equivalent to 20% of total blood volume resulted in rapid decrease in SBP by 60% (р ˂ 0.001), slight decrease in HR by 5–7% (р ˂ 0.05), while RR remained unchanged (р ˃ 0.05). In groups 1 and 3, when Tr 34 °С (referred to as baseline) was achieved by the beginning of monitoring, SBP was 11% (р ˂ 0.05) and 30% (р ˂ 0.001) lower than normal, respectively, HR decreased by 16–17% (р ˂ 0.01) in both groups, while RR decreased by 5% (р ˃ 0.05) in group 1 and 24% (р ˂ 0.01) in group 3. In group 2, when monitoring was begun (baseline), SBP increased by 29% (р ˂ 0.01) of minimum SBP measured during blood collection, HR and RR slightly decreased (р ˃ 0.05), and Тr was 37.1 ± 0.2 °С. Thus, by the beginning of monitoring (baseline) the animals’ physiological parameters reached certain levels that were different from normal, after that the required for the experiment Tr of 34 °С was maintained in animals of groups 1 and 3 for 3.5 h, and animals of group 2 were kept at room temperature.
In group 1 (fig. 2) in the 15th minute of hypothermia the rat’s SBP slightly increased to 107.7 ± 5.7 mmHg (р ˂ 0.05), but later it was within the range of 85–98 mmHg. HR of animals in this group was slightly higher compared to baseline within the first 1.5 h of monitoring, then in was at the baseline level, but did not increase to normal. The pairwise comparison of RR in group 1 revealed slight significant differences between the normal value and the values reported in animals with hypothermia (р ˂ 0.05) throughout the experiment.
In group 2, Tr decreased throughout the experiment and reached 34.3 ± 0.3 °С by the end of monitoring (after 3.5 h). SBP grew evenly within 1 h to reach 80 mmHg (р ˂ 0.01), after that it remained at this level till the end of monitoring. HR showed a downward trend throughout the period of monitoring, in the end of this period HR was 16% lower than normal and 9% lower than baseline (р ˂ 0.01). RR was at the baseline level.
In group 3, SBP increased to 53.9 ± 3.7 mmHg by the beginning of cooling, it continued raising to 72.4 ± 4.7 mmHg at the point corresponding to the beginning of monitoring during further cooling and reached its maximum (92.1 ± 4.2 mmHg) after blood loss on the 15th minute of monitoring (р ˂ 0.001).
HR and RR were at the baseline level throughout the period of monitoring.
Fig. 3 (fig. 3) provides the results of the direct measurement of pial artery diameter in µm.
During the stage of experiment between the animal’s initial state and the baseline values reported at the beginning of monitoring the changes in diameter of blood vessels were calculated as a percentage of the initial diameter (normal). Vasoconstriction was observed in all three groups during both preliminary blood exfusion and cooling of animals. At the moment when SBP reached its minimum constriction of blood vessels was 9% in group 2 and 4% in group 3. After the animals were cooled by the beginning of monitoring, the decrease in the diameter was 17.2 ± 2.8% of normal in group 1 and 18.4 ± 2.3% in group 3 (р ˂ 0.001).
The diameter of blood vessels reported at the beginning of this stage (baseline) was considered as 100% to objectively assess subsequent microcirculatory bed changes during the experiment. These changes are shown in fig. 4. Persistent hypethermia (group 1) was associated with initial decrease in the diameter followed by the diameter restoration to the baseline level within 2 h. In group 2, the experiment involving monitoring at room temperature showed that the diameter of cerebral microvessels continued to decrease within the first hour and then remained at the level of 80% of baseline showing no trend towards restoration. Constriction of the arteries in animals of group 3 that experienced both blood loss and hypothermia was 2 times lower than in animals of group 2 in the first 45 min of monitoring, later this ratio increased.
DISCUSSION
The major factors of the acute hemorrhage syndrome include reduced total blood volume, changes in blood vessel tone and cardiac output reduction [7, 24]. It is believed that blood loss that constitutes 15% of total blood volume triggers a compensatory mechanism, however, this mechanism turns out to be untenable in terms of preventing progressive hypotension. Our study involved the use of the small animal model of moderate blood loss (average exfusion rate 0.36 mL/min) with the total blood loss of 20% of total blood volume that resulted in the SBP decrease to ≈ 40 mmHg. Later, slight blood pressure compensation was observed in normothermia: in group 2, SBP increased to 55 ± 3.5 mmHg by the beginning of monitoring and then gradually increased with time to reach 70–80 mmHg. Hypothermic exposure along with blood loss (group 3) resulted in the earlier and larger (up to 90 mmHg) compensatory increase in SBP. Such results suggest that in the conditions of acute blood loss that constituted 20% of total blood volume SBP compensation was achieved faster and more effectively in animals with mild hypothermia than in normothermic animals.
Undoubtedly, blood pressure is merely a means of the tissue energy supply and does not fully reflect the tissue hypoperfusion associated with acute loss of a portion of total blood volume. However, the state of tissue circulation can be roughly estimated based on the SBP changes. It is believed that capillary blood flow is preserved when SBP exceeds 80 mmHg, SBP below 55 mmHg is associated with the loss of organ blood flow autoregulation, and critical cerebral perfusion impairment occurs when SBP is below 35 mmHg [24]. In our study involving the use of hypothermia a faster SBP compensation to до 90 mmHg was observed, then SBP was maintained within the range of 75–80 mmHg. That is why it can be assumed that mild hypothermic exposure prevents or significantly reduces the risk of organ hypoxia.
The findings of our experimental study confirm that mild hypothermia with or without moderate blood loss definitely affects the changes in the state of microcirculatory bed in anesthetized rats. We revealed vasoconstriction relative to baselines in animals of all experimental groups (fig. 4). Only slight differences in HR and RR throughout 3.5 h of monitoring were reported in animals of different experimental groups in the first 90 min, and in the final phase the values of these indicators were the same (p > 0.05). Furthermore, the differences in SBP between all groups by the end of monitoring were nonsignificant. Thus, it was shown that the 3.5 h exposure in hypothermic animals, normothermic animals with blood loss, and animals that experienced the combined effects of blood loss and hypothermia did not lead to significant differences in SBP, HR and RR in the final phase, but were associated with the changes in the diameter of pial arteries in animals of all studied groups.
The compensation to blood loss results also from the reflex sympathetically mediated arteriolar vasoconstriction induced by catecholamines acting on the α1 receptors [7]. Our study has shown that the spasm occurs not only in the peripheral, but also in the cerebral arteries, at least in the parietal cortex we have studied. It is believed that the key role in redistribution of blood needed to provide adequate blood supply of the brain tissue is played by the pial arteries [25].
We revealed microcirculatory disorders associated with hypothermia that took place since the very beginning of cooling. This was in line with the existing studies. Thus, when studying the effects of moderate hypothermia on the dynamics of microcirculatory bed in the zone of the tail vein projection in rats, vasoconstriction and reduced indicators of microcirculation were revealed [26]. The experiments on the cerebral arteries showed the same relationship: blood flow in the cerebral microvessels progressively decreased during cooling [21]. Microcirculation can play a crucial role when the cell metabolism adjusts to the temperature fluctuations. The decrease of the temperature, and, therefore, metabolic rate, results in the constriction of precapillary resistance vessels, thereby reducing blood flow. This results in the less effective perfusion capability of microcirculation [20, 21]. However, these studies show that cooling did not lead to poorer supply of oxygen to the brain, since the oxygen concentration was stable or even elevated when the cerebral artery perfusion was reduced. This important fact suggests that the ratio of the need and oxygen supply to the brain is improved in hypothermia.
The relationship between hypothermia and hemorrhage was studied repeatedly under different conditions, however, the number of studies focused on assessing the impact of the systemic hypothermia effects on the cerebral microcirculatory bed in acute blood loss is low. The hemorrhagic shock models of different animal species have demonstrated improved survival associated with hypothermia [8–13]. The improvement of blood flow through cerebral microcirculation by means of mild hypothermia during cardiopulmonary resuscitation has been reported [18, 19, 27]. Our study has also shown that the use of mild hypothermia improves the state of the rats’ cerebral cortex microcirculatory bed after moderate acute blood loss. This is consistent with the findings of the studies showing that systemic hypothermia provides protection against further impairment affecting microcirculatory beds of the kidneys, intestinal villi, and the sublingual space in hemorrhagic shock [22].
In our previous study, when the animals were subjected to continuous immersion cooling until the hypothermia-induced respiratory arrest was achieved, constriction of blood vessels by ≈ 20% of normal was observed during the period of deep hypothermia, while in animals with previously evoked blood loss (35% of total blood volume) that were further cooled constriction of blood vessels exceeded 30% [23]. In this study we did not use cooling to such low temperatures: animals of groups 1 and 3 were cooled only to Tr 34 °С, and blood exfusion was 20% of total blood volume. Constriction was less severe under such mild conditions, which was indicative of the direct relationship between the degree of vasoconstriction and the degree of the body’s exposure to negative factors.
Neuroprotective mechanisms of hypothermia used after the cerebral microcirculation impairment are poorly understood. After reperfusion injury, mild hypothermia reduces the increased cerebral oxygen extraction ratio [19]. The review focused on the effects of therapeutic hypothermia in neurological disorders reports that reduced metabolic rate and the decrease in the brain’s need for oxygen and glucose are the main mechanism underlying protective effects of hypothermia [28]. It can be assumed that it is the reduced need for oxygen that underlies compensation of reduced blood flow by hypothermia after the acute hemorrhagic exposure.
CONCLUSIONS
The findings suggest that mostly vasoconstrictor effects were observed in the pial microvessels of anesthetized rats under the conditions of mild hypothermia, hemorrhage constituting 20% of total blood volume or combined exposure to both. The use of mild hypothermia led to the significantly decreased vasoconstrictor effect of moderate acute blood loss on the cerebral microvessels. It should be noted that the current data on the use of hypothermia in the models of animal or human injury or hemorrhage are scarce, despite the large number of studies focused on the use of hypothermia in medical practice. Further research is needed to determine the possibility and most effective schemes of using hypothermia in injuries and hemorrhage.