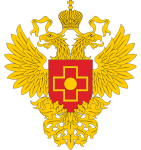
REVIEW
The effect of moderate and low doses of ionizing radiation on higher nervous activity of humans and animals
Ural Research Center for Radiation Medicine, Chelyabinsk, Russia
Correspondence should be addressed: Natalia I. Atamanyuk
Vorovskogo, 68A, Chelyabinsk, 454141, Russia; ur.liam@arhlup_ativ
The factors currently shaping the problem of radiation protection of people are the increasingly widespread introduction of nuclear technologies, planning of interplanetary flight programs, sharply increased risk of use of nuclear weapons and "dirty" bombs, need to protect healthy organs and tissues of patients during radiation therapy, and improving availability of nuclear medicine.
Traditionally, brain has been considered a fairly radioresistant organ. However, several animal and human studies [1-3] have presented accumulated data that demonstrates molecular genetic, morphofunctional, physiological changes in the brain, changes in higher nervous activity (mainly cognitive dysfunction, anxiety and depressive disorders) caused by moderate and low doses of radiation [1–3]. The 2012 UNSCEAR report defines doses below 0.1 Gy as low, doses from 0.1 to 1 Gy — moderate, if radiation is sparsely ionizing, and doses above 1 Gy — high [4].
The reports of both ICRP and UNSCEAR [5, 6] analyze reaction of the central nervous system to radiation exposure in the context of medical procedures, radiation accidents or work with ionizing radiation sources, with the considered consequences being higher risk of brain tumors and cognitive dysfunctions. The 2019 Report by the National Council on Radiation Protection & Measurements [7] contains the most complete summary of information about the effect of ionizing radiation on the central nervous system. The primary purpose of this report was to look into all possible aspects of the effect of cosmic radiation on brains and higher nervous activity of astronauts.
Understanding how low doses of ionizing radiation received by the brain affect cognitive and behavioral capabilities and patterns is essential for ensuring human radiation safety in cases of medical exposure, occupational exposure, including that associated with space missions outside the Earth's magnetic field, as well as radioactive pollution of the environment. The epidemiological and experimental data describing the effect of low doses, the possible chronic exposure threshold value after which radiation begins to compromise functions of the central nervous system, remain quite limited and contradictory. Thus, it is possible to formulate a research problem about the cause-andeffect links between radiation exposure and development of early and long-term adverse effects on the part of the central nervous system (disorders of mental functions, neurodegenerative diseases, brain neoplasms). This review summarizes the currently available scientific data on this problem.
Epidemiological data
Overall, large doses of ionizing radiation received by the brain are an established risk factor promoting development of neoplasms and cognitive impairments. The latter, as a rule, has ties with deficiencies in hippocampus-dependent processes: verbal-semantic and spatial memory, learning, spatial information processing; such impairments develop in the long term after irradiation [8]. The causes of these long-lasting disorders are impairment of neurogenesis and oligodendrogenesis in the subependimal and hippocampal regions; blood-brain barrier deficiency; ablation of capillaries and damage to the microvascular endothelium [9], sustained activation of immunocompetent microglial cells and increased level of proinflammatory cytokines [10].
According to the 118 ICRP report [5], the threshold dose values for development of cognitive disorders in adults are 1–2 Gy, and for children exposed at the age under 18 months it is 0.1 Gy. The effect of low doses of ionizing radiation on young children in terms of subsequent decline of their cognitive capabilities was first shown on a Swedish cohort that received 100–250 mGy in the context of treatment of cutaneous haemangioma [11]; the researchers have proven the dosedependent learning and logical thinking disorders suffered by the people from that cohort in adult age. Examination of people exposed to radiation at the prenatal stage as a result of contamination of the Techa river has shown that the respective group includes more subjects with non-psychotic mental impairments than the control group, the difference statistically significant. The prevailing disorders were of organic nature, i.e., cognitive and asthenic. The mean red bone marrow dose registered in the exposed group was 0.09 Gy, as calculated for the red bone marrow of a fetus [12]. However, authors of a systematic review [13] that included epidemiological studies published before 2018 have claimed insufficiency of the data to allow a reasoned conclusion about any effect on development of the central nervous system that low and moderate doses of ionizing radiation may have if received during prenatal life, childhood or adolescence, although they acknowledged the limited evidence of relationship between low and moderate doses and decline of the general cognitive and language capabilities.
The question of how ionizing radiation affects the risk of dementia and neurodegenerative disorders remains ambiguous. Examination of a cohort of employees working at the nuclear production cycle of Mayak Production Association (town of Ozersk, Chelyabinsk Region) has uncovered a linear relationship between the incidence of Parkinson's disease and the cumulative dose of gamma radiation, the findings confirmed after adjustment for gender and age [14]. Examination of a cohort of French nuclear power industry workers has shown a statistically significant link between the risk of dementia and Alzheimer's disease and the received dose of ionizing radiation, but authors of the report urge caution in interpretation of these results [15]. In a Japanese cohort of survivors of the Hiroshima and Nagasaki bombings comprised of those who received doses of radiation in childhood (0 to 4 Gy), the decline of neurocognitive functions correlated with age, without an identifiable link to the dose [16]. Another study of the same cohort did not reveal any increase in dementia incidence associated with the exposure to radiation [17]. A 2022 metaanalysis has shown a dose-dependent increase of risk of cerebrovascular diseases and Parkinson's diseases, as well as death therefrom, associated with low and moderate doses received in adulthood [18].
In a study involving Chernobyl nuclear accident liquidators, Loganovsky et al. note high incidence of cerebrovascular diseases, organic psychic and depressive disorders, cognitive impairments and dementia, the numbers climbing up with the dose received; some effects were found to manifest at the dose of 50 mSv and above [19]. Any analysis of data on the effects of radiation on population exposed thereto in the context of a nuclear accident should factor in the stress associated with the very fact of the exposure, which, regardless of the actual excessive background radiation or lack thereof, may up the frequency of psychic disorders [20]. Also, there are some difficulties in establishing causal relationships between radiation and psychoneurological effects in the context of analysis of epidemiological data: firstly, it is important to have a comparable control group (living standard, frequency and quality of medical care) when processing the data describing exposed population; secondly, investigations involving professionals should factor in conditions of health screenings preceding their admittance to work with sources of ionizing radiation; and thirdly, disease diagnosing and symptom interpretation activities should follow uniform standardized approaches.
As for the capability of low and moderate doses of ionizing radiation to increase the risk of neoplasms, a meta-analysis of studies published before 2022 revealed no links between such doses and the risk of CNS tumors in adults [21]. However, a 2022 paper summarizing assessment of risk of cancer and benign CNS tumors after exposure to X and Y rays (regardless of the source) during prenatal period or childhood, the doses being low and moderate, has shown an excessive risk of neoplasms associated with doses below 0.1 Gy, with the threshold value decreasing to 0.02 Gy for those exposed to background radiation from radioactive precipitation or medical equipment while in the mother's womb [22].
Experimental data
The data from experimental animal studies are much more abundant. Such work aims to establish the causal relationships and molecular and cell mechanisms behind the effects of radiation on the CNS. Mice are considered a representative model for investigation of radiation-induced effects on brain and violations of its development when the received dose is clinically significant, although animals cannot be said to allow a full reproduction of the cognitive alterations registered in human beings [23].
In animals, a one-time exposure to ionizing X rays, Y rays, or stream of protons or heavy ions that simulates cosmic radiation, compromises cognitive functions of learning, spatial memory, exploratory activity, and ups the anxiety level [2, 3, 24, 25]. There is a wide range of behavioral tests designed to examine these parameters.
There is a generally accepted concept that puts death of neural stem cells and impaired neurogenesis [26–29], coupled with microglia's pro-inflammatory activation [26, 30], behind radiation-induced violations of mental functions. The process involves release of pro-inflammatory IL1β, TNFα and IL6 cytokines, increased expression of the genes of CCL2 (macrophage chemoattractant) and growing numbers of CD68+ macrophages [31]. The weakening of the inflammatory response caused by the CCR2 receptor gene mutation supports preservation of the background number of neuronal BrdU+ progenitor cells [31].
Under normal conditions, neurogenesis occurs in three regions of an adult brain: dentate gyrus, subventricular region and cortex cerebelli. It is the response of neuronal progenitor cells to radiation and altered neurogenesis in the hippocampus region that at least contributes to, if not causes, radiationinduced memory disorders and cognitive impairments that persist for some time after exposure. Various animal models were used to study radiation-induced changes in neurogenesis depending on the dose, fractionation regime, time after exposure and age at the time of exposure [27]. A targeted irradiation of the dentate gyrus of newborn mice at the dose of 1 Gy, as opposed to a full-body irradiation at the same dose, leads to alterations in cell differentiation 3 months after exposure (the shares of BrdU+/NeuN+ cells go down, share of BrdU+/GFAP+ goes up), and some deterioration of the animals' spatial memory [28].
The most pronounced effects are seen following irradiation during embryonic development or neonatal periods. There are periods of brain development that are critical for its normal maturation. In many mammalian species, this is the time of perinatal development, but in case of mice and rats, such critical period extends to the first 3–4 weeks of life. Mice, in particular, are particularly vulnerable to radiation on days 3 through 10 after birth [29, 32].
One-time moderate dose during embryonic development or neonatal period changed mice's spontaneous behavior in the new environment in their adulthood; these persistent changes indicated compromised ability of mice to integrate sensory information into motor production [26, 32], impaired spatial memory and ability to learn, increased anxiety level [33, 34], altered social behavior [34], modified ability to explore and adjust to a new environment [26, 35]; motor activity and coordination were also disrupted [34]. In different papers, the age of manifestation of such behavioral changes ranged from 4 to 15 months.
Hippocampal neurogenesis defects persist and accompany changes in the cognitive functions that appear in the long term period after exposure [26]. The ratio of pools of maturing neurons changes: in the early stages, the proportion of radial glial-like GFAP+ cells increases and the share of proliferating PCNA+ progenitor cells decreases, followed by a compensatory growth of the PCNA+ cells and progenitor neurogenic type-2 cells Sox2+, which further leads to a drop in the density of mature granule neurons NeuN+ and proliferating PCNA+ cells up to 6 months after exposure [29]. Microglia also becomes more active: the number of Iba1+ cells and GFAP+ astrocytes increases, and their morphology changes; there appear more postsynaptic protein PSD-95 and MAP-2 because of the malfunctioning Rac1-cofilin signaling pathway in the hippocampus and cerebral cortex [26, 29], while the level of tau protein there grows up [35] and the number and complexity of myelinated axons goes down [36]; the density of microvessels changes, and the drop in the activity of most proteins needed for production of ATP disrupts the function of mitochondria [29]. Some evidence suggests that the changes in neurogenesis and neuroinflammation may disappear earlier than the changes in animal behavior [33]. There is also evidence of an opposite situation when the number of activated microglia cells in the hippocampus remains increased and the number of astrocytes decreased in the term of up to 24 months, when behavioral deviations cease to manifest [30].
For most of the described effects, the early-age acute irradiation threshold dose is 0.3–0.5 Gy [32, 34]. However, some changes are induced with lower doses. Acute irradiation at the dose of 0.1 Gy raised the expression of proinflammatory cytokines in the hippocampus [31]. Having received a dose from 0.1 Gy prenatal, at the age of 4–15 weeks mice were more active socially in tests assessing social memory and sociability, and the Morris water maze test revealed dose-dependent changes in behavior strategies that signal of spatial memory disruptions [34]. A dose of 0.1 Gy received by mice on the 10th day after birth caused a small but persistent (registered for 6 months) depletion of the pool of proliferating cells in the dentate gyrus: the number of Sox2+ type-2 cells increased 1 week after irradiation and the number of proliferating PCNA+ cells decreased after 6 months. In case of adult mice, same dose increased the density of mature NeuN+ neurons after 6 months. At the same time, the signs of mitochondrial homeostasis activation were more vivid in the animals irradiated at the age of 10 weeks, while the area of microvessels in the hippocampus of these subjects was found to diminish, which indicates a possible protective effect of a 0.1 Gy dose that depends on the age at the time of exposure [29].
A single 1 Gy dose of Y rays given to 10-days-old mice translated, in their adulthood, into a significantly smaller number of GFAP+ type-1 stem cells with radial glial-like morphology in the subgranular zone of the dentate gyrus, while a dose from 0.5 Gy caused a dose-dependent drop in the proliferating PCNA+ cells, and a dose from 0.1 Gy triggered a dosedependent decline in the proliferating Ki67+ precursors, which means disruption of neurogenesis in this area of the brain in adulthood [26]. The described changes concerning stem and proliferating cells led to a dose-dependent decrease in the number of mature neurons in dentate gyrus: by 21% for a dose of 0.1 Gy, by 26% for a dose of 0.5 Gy, and by 37% for a dose of 1 Gy [26].
In another study, mice were irradiated while newborn, and examination of proteome of their hippocampus and cerebral cortex 7 months after irradiation revealed a dose-dependent increase in the number of deregulated proteins for the doses from 0.02 to 1 Gy, and the doses of 0.5 and 1 Gy produced different profile of proteins with impaired regulation than lower doses [26].
In the course of 2 years, a one-time exposure of 10-week-old mice to Y rays (doses 0.063 Gy, 0.125 Gy, 0.5 Gy) triggered the following changes in sensorimotor and locomotor parameters: at the age 4 months, the animals that received 0.5 Gy had a decreased acoustic startle response; at the age of 12–18 months, the speed of movement decreased, same as the total distance traveled in the open field test; and a dose of 0.063 Gy, on the contrary, led to a slight improvement in the sensorimotor reaction and exploratory behavior at the age of 18 months [30]. However, only the doses of 0.125 Gy and 0.5 Gy (but not 0.063 Gy) caused manifestation of quantitative and morphological signs of the increased immune activity registered at the age of 24 months. In the cases of mice irradiated at 0.125 and 0.5 Gy, examination of morphology revealed a smaller number of GFAP+ astrocytes in the dentate gyrus, and these astrocytes had fewer endings and nodes, and decreased branch length. A dose of 0.5 Gy increased the number of Iba1+ microglial cells, but the cells had an amoeba-like shape and fewer endings, nodes, intersections, with shorter branch length. The dose of 0.063 Gy, on the contrary, produced a neuroprotective effect at the 24-month mark: hyper-ramification of microglial cells and astrocytes was noted [30].
In another study, researchers report an opposite situation: they examined mice that received 0.1 Gy while newborn 6 months after irradiation and registered a significant increase in the number of GFAP+ astrocytes in the hippocampus hilus, while larger numbers of CD11b+ cells of activated microglia and greater concentration of pro-inflammatory cytokine TNF in the hippocampus were observed only in the subjects irradiated at 1 Gy [26].
Irradiation with Y rays in the range from 0.05 through 0.5 Gy revealed a dose-dependent change in the number of apoptotic cells, but proliferation arrest of neuroblasts required a threshold dose of 0.2 Gy (and, as the dose increased, the number of Ki67+ cells decreased and the time of proliferation arrest increased), while the dose of 0.5 Gy decreased the proportion of immature Dcx+ neurons [37]. The dose of 0.01 Gy triggered a slight decrease in the expression of TSPO in the endothelial cells of hippocampus vessels and in the ependymal cells in the short term after irradiation; higher doses (up to 2 Gy) brought no noticeable changes [38].
There are other adverse factors that may play an important role in the combined effect together with the low doses of radiation: for example, a single dose of 0.1–0.2 Gy of ionizing radiation coupled with ketamine in 10-day-old mice led to cognitive impairments in their adulthood, which was not the case for radiation alone [39].
Overall, the results of experimental and epidemiological studies summarized in the review [3] confirm capability of low doses of ionizing radiation to adversely affect cognitive function. It is important to factor in age both at the time of exposure and at the time of examination, since some effects appear immediately after irradiation and gradually fade away, while other effects develop over time.
There are many works investigating the effects of cosmic radiation simulated with heavy particles. One of them has shown the probability of alteration of cognitive functions to be dose-dependent in case of exposure to 28Si or 56Fe with doses ranging from 0.01 to 0.1 Gy [40]. High-energy particles and neutrons radiation exposure was found to cause effects similar to those produced by the Y rays: suppression of neurogenesis in the hippocampus region [41], long term persistence of signs of microglia activation [42], disruption of the synaptic transmission in hippocampus region [43], disruption of the functional links between hippocampus and peripheral cortex [42], decline of the mitochondrial functions and changes in the expression of a number of proteins [44]. As a result, animals that received doses in the range from 0.05 to 0.6 Gy exhibit certain cognitive dysfunctions both in the short and long term periods after irradiation: deteriorating ability to recognize and switch attention, impaired spatial memory, episodic memory, executive function deficiency, increased anxiety level [45]. In some cases, behavioral changes were detected 12–15 months after irradiation [42].
Different cognitive functions are mediated by different brain structures and may have different sensitivity to radiation. For example, irradiation with 56Fe particles enables male C57Bl mice to better solve a hippocampal-dependent task (discrimination learning) without changing the effectiveness for a striatum-dependent task (rule-based learning) [46].
There is much less experimental data on the effects of chronic or fractionated radiation. Most studies involved a single session of acute irradiation, while clinically and environmentally significant situations mainly imply chronic or fractionated exposure.
Mice chronically irradiated with a neutron-photon mixed field at a dose of 0.18 Gy (dose rate — 1 mGy/day) showed decreased excitability of hippocampal neurons and impaired long-term potentiation of hippocampus and cerebral cortex, learning and memory disorders [47], as well as impaired synaptic plasticity in the hippocampus-prefrontal cortex axis [48]. Fractionated X ray irradiation at a cumulative dose of 0.5 Gy makes behavior more anxious and causes changes in motor activity [49], as is the case with acute exposure.
Probably, the effects of chronic and fractionated irradiation in general are also based on the disruption of neurogenesis (as acute irradiation at a dose of 2 Gy or fractionated irradiation at the same cumulative dose) [50], impaired synaptic transmission, decreased number of synapses and changes in the electrophysiological parameters of the hippocampal and cortical neurons [47, 48, 51], as well as activation of microglia [52]. It has been shown that fractionated exposure to low doses of ionizing radiation causes dose-specific changes in the global genomic methylation of different regions of a mouse's brain against the background of changes in the emotional state and increased anxiety, impaired coordination of movements in walking [49]. Compared to fractionated irradiation (20 fractions, 0.1 Gy each), acute irradiation with Y rays at a dose of 2 Gy triggered a more pronounced increase in the number of activated microglia cells and a decrease in the number of neuronal progenitor cells [52]. However, chronic bombardment with heavy particles (doses of 0.4–0.5 Gy) that simulated galactic cosmic radiation, had a more pronounced effect on the change of electrophysiological properties of hippocampal pyramidal cells in mice than acute irradiation [51]. Fractionated exposure to Y rays translated into damage to DNA in the tissues of frontal cortex and cerebellum that increased after each fraction, although in the hippocampal tissue, this indicator has grown only after the first fraction [49].
The available publications contain no data on the experiments designed to assess the effect of chronic or fractionated irradiation at low doses (cumulative dose up to 0.1 Gy). There are confirmed effects of acute irradiation at low doses and the comparable effects of fractionated and acute irradiation at moderate doses, but these do not eliminate the possibility of reaction from the CNS to chronic exposure at low doses. According to the report by UNSCEAR [53], such exposure causes changes in the immune system regulation mechanisms, and one of the described effects of ionizing radiation on CNS is pro-inflammatory activation of microglia. Other known effects of low doses include cellular-level DNA damage, unstable chromosomal aberrations, apoptosis of the most radiosensitive cells [54], which can potentially affect neurogenesis, especially in the critical periods of development of the brain.
Experimental studies have shown that in some cases, gender conditions the effect of exposure: for example, irradiation with 28Si slows neurogenesis in the long term, but this is a male-only effect, females remain unaffected [41]. In a study that simulated acute or chronic galactic radiation, the "object in updated location" test revealed persistent disruptions in formation and reconsolidation of hippocampal-dependent memory in female mice but not in male, while female subjects were better at recognition of the new objects than male, and only the chronically irradiated males showed increased aggressiveness in the tube dominance test [51].
Some experimental data support the hypothesis about the potential capability of ionizing radiation to increase the risk of neurodegenerative changes. Inadequate chronic activation of microglia is observed both after irradiation and in cases of depression, Alzheimer's disease, Parkinson's disease [55]. Ionizing radiation at low and moderate doses can trigger molecular mechanisms that support development of the Alzheimer's disease [29], such mechanisms associated with oxidative stress, increased number of amyloid plaques in the brain [24], and accumulation of the tau protein [35]. In mice with ApoE deficiency used as a model of Alzheimer's disease, chronic irradiation for 300 days at cumulative doses of 0.3 Gy and 6.0 Gy caused changes in the number of proteins associated with control of synaptic plasticity, calcium-dependent signaling and brain metabolism [56]. However, there were also differences: signaling of the Rac1-cofilin pathway was activated only at a lower dose of 0.1 mg/day, and at the same level of exposure the amount of activated microglia in the hippocampus and expression of TNF and lipid peroxidation decreased. Thus, several molecular targets for low dose chronic radiation overlap with those in Alzheimer's pathology [56].
CONCLUSION
Currently, there is an extensive amount of information about the effect of ionizing radiation on the brain and higher nervous activity. High doses of ionizing radiation are an established risk factor for cognitive impairment, but the results of epidemiological studies assessing the effect of moderate and low doses on the human CNS are ambiguous.
Experimental animal studies have revealed such effects of irradiation as disruption of the learning and memory functions, increased anxiety level, and locomotion disorders. It has been established that the main causes of development of radiationinduced effects on the part of the CNS upon exposure to sparsely ionizing radiation at doses above 0.5 Gy are violation of normal neurogenesis and suppression of proliferation of neuronal stem cells, as well as pro-inflammatory activation of microglia. Other identified effects include violation of the blood-brain barrier permeability, changes in the synaptic transmission, balance disorders of neurotransmitters, etc. Active stages of brain formation during intrauterine and early postnatal development are the periods when ionizing radiation can do most damage. Then, the threshold dose level for acute exposure to Y rays is 0.2–0.3 Gy.
There are significantly less data on the effects of low doses of radiation and effects of fractionated and chronic exposure. The available papers show that acute irradiation at doses of about 0.1 Gy can have a multidirectional effect, producing both mild adverse effects on higher nervous activity and the brain, and a neuroprotective effect, depending on the age at the time of exposure, time after exposure and other factors. Fractionated X ray irradiation at the doses from 0.5 to 2 Gy can also trigger changes in the higher nervous activity; as for the potential effects of prolonged exposure to low dose ionizing radiation, there are no experimental data.
For the purposes of improving radiation safety and development of pathogenetic means of prevention and treatment of radiation-induced CNS disorders, further research efforts should be aimed at studying chronic exposure and assessment of both the functions of the higher nervous activity and morphofunctional indicators of the brain, as well as establishing causal relationships between chronic exposure and its adverse effects on the part of CNS in the long term post-exposure.