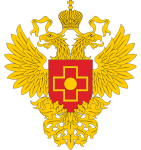
ORIGINAL RESEARCH
Specifics of reaction of human cardiovascular system to immersion in cold water
1 Saint Petersburg State University, St Petersburg, Russia
2 Lesgaft National State University of Physical Education, Sport and Health, St. Petersburg, Russia
3 Federal Research and Clinical Center for Sports Medicine and Rehabilitation of the Federal Medical Biological Agency, Moscow, Russia
Correspondence should be addressed: Mkrtych G. Ogannisyan
B. Dorogomilovskaya, 5, Moscow, 121059, Russia; ur.abmftrops@gmnaysinnago
Funding: the study was conducted as part of discharging work duties at the St. Petersburg State University.
Author contribution: Baranova TI — article conceptualization, authoring, general editing; Rybyakova TV — article conceptualization, general editing; Dmitrieva MO — search for and analysis of sources, statistical data processing; Anisimov DA — search for and analysis of sources, compilation of tables, preparation of figures; Tarasova MS — search for and analysis of sources, article authoring; Ogannisyan MG — selection of approaches to mathematical modeling and their optimization.
Compliance with the ethical standards: the study was conducted in accordance with the Helsinki Declaration and approved by the Human Research Ethics Committee (Minutes #40 of March 07, 2012). All subjects were familiarized with the test protocol and signed an agreement to participate therein.
In 2022, winter swimming was included in the Russian National Register of Sports. Extreme cold stress is inherent in this activity, which makes it significantly different from classical and open water swimming. The said stress also requires the practicing athlete to have higher functional reserves that should be evaluated and controlled.
There are two body temperature maintaining mechanisms triggered upon immersion in cold water without special equipment, one boosting bodily heat production and another limiting heat release [1, 2]. Such an act also activates reflective defense mechanisms: controlled by the sympathoadrenomedullary system, peripheral vessels spasm, blood flow in skin and superficial muscles slows down (thus the output of heat is restricted), liver releases glucose, fat depots mobilize fatty acids, brown adipose tissue starts generating heat [3–5]. When the face is in the water, the body triggers diving reflex to save oxygen, and parasympathetic stimuli from cold receptors (mainly in facial skin) running along the vagus nerve's cholinergic pathway to the heart's sinus node intensify. Heart rate slows down, since the said reflex has a strong arrhythmogenic effect [6-8]. Further aggravation of the cholinergic stimuli affecting airways can lead to bronchoconstriction, which entails the risk of lung ventilation impairment [9].
Given the above, it should be noted that swimming in cold and ice-cold water is a rather common activity, which does not always trigger adverse reactions on the part of the cardiovascular system [10]. We believe that the negative reactions of the body that threaten human life when immersed in cold water may stem from high reactivity of the efferent part of reflex response. There are several factors that can make said reactivity high; for example, beginner cold swimmers may experience it because their bodies are unaccustomed to extreme cold, and other reasons may be rooted in the peculiarities of vegetative regulation formed during postnatal development, as well as in a person's genetic makeup [11–15].
This article analyzes the results of the earlier studies investigating the effect of cold water on adaptive reflex reactions, and, thereupon, seeks to characterize individuals at risk of a negative (health- and/or life-threatening) response to cold water immersion on the part of cardiovascular system.
The purpose of this work was to identify the specifics of adaptive cardiovascular reactions and assess the possibility of pathological abnormalities in the individuals exhibiting different vegetative reactivity of cardiovascular system to cold-hypoxia test (CHT) and cold water immersion in the context of swimming.
METHODS
We have summarized two types of research activities, laboratory and field. The first part presents materials from laboratory studies that involved triggering of the diving reflex by immersion of face into cold water, the cold-hypoxia test (CHT). We assessed arrhythmogenicity of the said reflex in people with different reactivity of the cardiovascular system to a cold stimulus.
The second part gives results of field studies, which took form of relay and competitive swims in water of different temperatures (+7–9 °C, +16–17 °C, and +1.5–2.5 °C). The analyzed factors of the cardiovascular system's reaction to swimming in cold water are myocardial conduction, blood pressure (BP), and heart rate (HR).
The study was conducted in 2008-2023, on the basis of St. Petersburg University, in the Systemic Adaptivity Laboratory. The participants were 205 male and 255 female individuals, all practically healthy, aged 18–25 years, whose cardiovascular system exhibited no pronounced abnormalities. Those with a history of sinus node dysfunction, stage II hypertension, atrial fibrillation were excluded from the study.
According to the survey, 15% of the participants were smokers with an average experience of 4.3 ± 1.7 years. As a rule, we asked all subjects to abstain from smoking and coffee for at least 2 hours before the test, which usually took place in the morning, from 10 a.m. to 12 p.m.
Laboratory part of the study
For the test, the subjects lied down on a couch, back up, in the most relaxed state. The test simulating diving involved immersing face in water. The water temperature was +10 ± 2.2 °C, the temperature of air in the room +21 ± 2.3 °C. As a rule, there were three immersions, all after an unforced exhale. The participants were not allowed to hyperventilate before the immersions. The rest period between the immersions was 2 minutes; as a rule, this time was sufficient for pulse to restore to its original rate. For the first immersion, the participants held their breath until they started to feel uncomfortable, and for the following immersions — for as long as they could.
To register response of the cardiovascular system, we used electrocardiography (ECG), photoplethysmography (PPG), and continuous blood pressure monitoring before, during and after immersion, while the participants were recovering. Peripheral vessels blood filling was indirectly determined by the amplitude of systolic wave in finger phalanges (ACB, pm), vascular tone — by the time of its propagation (VPRV, s). PPG was recorded and analyzed with the help of REAN POLY 6/12 rheographpolyanalyzer, modification 03, version Elite (Medikom-MTD; Russia). To continuously monitor blood pressure, we used Finometer® MIDI (Finapres Medical Systems; Netherlands). The device employed to register ECG was Poly-Spektr-8/E cardioanalyzer (Neurosoft; Russia).
To describe the nature of the heart's chronotropic function during CHT, we used the following indicators: latent time of development of reflex bradycardia — l, s; time of occurrence of the maximum cardiointerval during the test — tmax, s, bradycardia aggravation rate — V, severity of bradycardia — S.B. Earlier studies present a detailed description of the types determination method [16]; based on these indicators, we distinguished four types of response: highly reactive, reactive, areactive, and paradoxical (fig. 1).
To analyze the data, we used GraphPad Prism 8 package for Windows 10. Mann-Whitney and Kruskal–Wallis tests allowed establishing the significance of differences for unrelated variables and for related paired samples. To assess the significance of differences in samples with a normal distribution, we used Student's t-test and one-way ANOVA. Statistical significance was registered at p < 0.05. In small groups, we looked for significance of changes for each person, before and after the swim, with ECG registered for 5 minutes. After assessing normality of distribution, we applied Student's t-test.
Results
Analysis of cardiovascular system's response to CHT revealed a reflex-driven heart rate slowdown, narrowing of the peripheral vessels (fig. 2, fig. 3; tab. 1), and growth of the blood pressure (fig. 2, tab. 1).
In the first series of laboratory tests, which involved 460 subjects, we investigated chronotropic reaction of the heart to CHT. Based on the occurrence and rate of aggravation of reflex bradycardia (one of the diving reflex components) caused by CHT, the participants were distinguished into 4 types, according to the method we developed earlier [16]. The relative figures were as follows: 40% of the subjects belonged to the highly reactive type, 45% — to reactive type, 10% — areactive type, and 5% — paradoxical type. This typification by cardiovascular system's parasympathetic reaction to CHT was needed to understand whether it affects development of cardiac arrhythmias, and, conversely, what deviations from the normal can result in the participants from the paradoxical type group (predominance of sympathetic stimuli). This was necessary in order to assess the possible pathological abnormalities risk in people with various intensity of reflex parasympathetic and sympathetic stimuli sent to the myocardium when immersed in cold water.
Analysis of myocardial conduction during CHT in subjects with different types of reactivity
Analysis of the dynamics of changes of myocardial conduction indicators in response ot CHT revealed that in those of highly reactive and reactive type, reflex-driven parasympathetic stimuli not only slow down heart rate (statistically significant increases of RR intervals), but also alter the rate of atrioventricular conduction (PQ intervals increase). We observed CHT slowing down atrial conduction (PQ interval grows longer) in most participants belonging to the said type groups, but, as a rule, the registered PQ intervals in such cases were within the normal range. However, two individuals of the highly reactive type and three of the reactive type group had the PQ interval exceeding the norm, which indicates a delay in pulse conduction and partial atrioventricular blockade (tab. 2).
In participants of areactive type, CHT triggered no significant changes (tab. 4). In those of paradoxical type, against the background of CHT, RR, QT and TP intervals were decreasing, and QTC growing, which means that while HR accelerates, ventricular conduction is slowing down (tab. 2).
Analysis of CHT-associated changes of blood pressure showed that DBP and SBP increased significantly in all the participants (fig. 4, fig. 5). We identified subjects of the highly reactive type to have higher initial BP. Those with paradoxical type had the lowest SBP. Moreover, in participants from the highly reactive group, repeated CHT caused DBP to progressively increase, reaching 175/115 mmHg in some cases.
Thus, in the majority of participants with moderate reactivity, CHT triggers reflex changes of adaptive nature, but in some subjects with high reactivity it can provoke atrioventricular blockade, cause a slowdown in ventricular conduction (in some of paradoxical type), push blood pressure up with a vasospasm in the background. These are the findings from CHT conducted in comfortable conditions with minimum stress. If the immersion in cold is full, the associated stress can make such deviations fatal.
Effect of cold-hypoxia training on reactivity of cardiovascular system
In order to establish the effect of adaptation to cold and hypoxia on vegetative regulation, we conducted a respective 6-week course with daily sessions. After CHT and typification by reactivity, 40 selected participants were distributed into 4 type groups (n = 10). Every day, in the course of 6 weeks, they immersed their faces into water with the temperature of +8 ± 2 °C, 3–4 times, having made an unforced exhale. After the course, CHT revealed decreased reactivity, which manifested mainly in a longer latent time of reflex bradycardia development and its slower progression. Seven individuals out of 10 from the highly reactive group transferred to the reactive group, but 3 retained reactivity at high level. From the reactive group, 4 participants moved to the areactive group, from paradoxical — 2 to the areactive, 3 to the reactive group, and 1 retained the original level of reactivity.
The analyses of reflex constriction of peripheral vessels and blood pressure dynamics also showed that training decreased reactivity, which was reflected in a smaller growth of DBP during CHT. On the contrary, after the course, SBP increased significantly in the context of CHT (tab. 3), accompanied by an increase in pulse pressure, and, consequently, stroke volume of the left ventricle.
Thus, adaptation to cold-hypoxia effects decreases reactivity of the cardiovascular system somewhat. However, there were participants whose reactivity did not change after the course; the possible reasons behind this are their individual characteristics, including genetic ones [12–14].
Changes in myocardial conductivity caused by swimming in open cold water
We analyzed state of the cardiovascular system after two relay swims. The first swim from Elagin Island to Kronshtadt took place on October 20, 2019, when the water temperature was +7.5–9 °C. The distance was 25 km. Four experienced winter swimmers (aged 37–52 years) participated in the relay swim. As prescribed by the rules of the International Winter Swimming Association (IWSA), the participants swam without wetsuits. Each individual swim lasted 20 minutes, with 60 minutes of rest inbetween.
We recorded ECG 30 minutes before the swim and at the 30th minute of the post-swim recovery. Under the influence of cold and physical activity, two out of four athletes had significantly longer QTc interval, i.e., ventricular conduction slowed down in them (tab. 4).
The second relay swim took place at the Oreshek Kronstadt site on June 12–13, 2021. Same 4 swimmers participated therein. They covered 103 km in 22 hours and 16 minutes. The air temperature varied from +16 to 22.3 °C. The water temperature in the Neva river and the Gulf of Finland was +16–17 °C. The swims lasted for 30 minutes, the rest between them — 90 minutes. The analysis of ECG revealed a statistically significant growth of the QTc indicator in 3 out of 4 swimmers (tab. 5), while one athlete had it decreasing significantly.
After both swims, no swimmer exhibited significant changes in the BP at the 30th minute of recovery. Thus, in the context of the 2nd swim (t = +17 °C), before it the overall SBP was 119.4 ± 7.3 mmHg, DBP — 78 ± 4.5 mmHg, and post-swim SBP was 123.3 ± 8.5 mmHg, DBP — 73 ± 5.3 mmHg, respectively. However, control of the athletes' BP pressure dynamics (n = 17) short after swimming 200 m (at 3rd–5th minutes of recovery) at water temperature of +1.5-2.5 °C revealed significant changes. Overall, we registered significant growth of SBP in the group. At rest, SBP was 134.4 ± 6.1 mmHg, after swimming — 148.5 ± 5.2 mmHg (p < 0.05); DBP at rest = 79.8 ± 3.1 mmHg, after swimming — 91.3 ± 7.1 mmHg (p < 0.05). However, in some swimmers, the pressure changed only slightly, but in others, SBP grew up to 190 mmHg, and DBP to 120 mmHg. The data describing these individuals were excluded from the processed pool. After swimming 200 m, heart rate of the athletes increased: at rest it was 76.8 ± 4.4 beats/minute, after swimming — 98.1 ± 4.7 beats/minute (p < 0.01). For one participant, cold water swimming translated into heart rate slowdown: before the swim it was 88 beats/minute, after the swim — 64 beats/minute.
DISCUSSION
The analysis of cold-hypoxia tests conducted in the laboratory showed that even when it is only the person's face immersed in water, there occurs a complex of reactions that reflect considerable changes in the cardiovascular system's operation. The reason behind this phenomenon is the diving reflex [17–19], which includes a set of actions simultaneous with activation of the sympathetic and parasympathetic parts of the ANS that send signals to the myocardium. The total effect of stimuli acting on the sinoatrial node from n. vagus and postganglionic sympathetic neurons also depends on the background state of cells of the sinoatrial node, which is shaped by various neuropeptides secreted by vascular endothelium and cardiomyocytes. The magnitude of the cardiovascular system's response to cold water swimming varies depending not only on external factors, such as water temperature, degree of adaptation to cold, etc., but also on the individual characteristics of the body's vegetative reactivity. Diving reflex causes parasympathetic bradycardia, whereas cold stress activates sympathetic tachycardia. These effects, although different in nature, can lead to arrhythmias [7, 8], especially in people with pronounced diving bradycardia. Thus, in some individuals of the highly reactive and reactive types, reflex parasympathetic stimuli affecting sinus node of the heart, in the presence of a pronounced bradycardia, can cause atrioventricular blockade, when impulses cannot reach the ventricles from the atria, which pushes the PQ interval beyond the norm. In some people of the paradoxical type that react to CHT as if it were a stress (expanding sympathetic stimuli to the myocardium), intraventricular conduction deteriorates against the background of a shorter cardiac cycle, i.e., QTc exceeds the norm. This reaction is common for open cold water swims, registered, inter alia, among experienced swimmers adapted to cold water. These data confirm the current understanding that has the diving reflex possessing arrhythmogenic power [6–8]. In addition, these deviations may aggravate against the background of disruption of K+ metabolism associated with physical exertion during swims, as well as hypothermia while in the water and several minutes afterwards, when the body temperature continues to decrease, including that of the core. These factors may increase the risk of QTc reaching critical values that can lead to cardiac arrest [20, 21].
Peripheral vessels constrict in response to signals associated with the diving reflex that originate in the vasomotor center of medulla oblongata and move along adrenergic sympathetic fibers to the vessels' muscle walls. Some of the participants, mainly those of the highly reactive type, exhibit growth of BP progressing with each subsequent face immersion in cold water, which is associated with a slow recovery of tone of peripheral vessels that fail to return to their original state within a two-minute interval between dives. We observed a similar reaction in some winter swimmers, when successive swims in cold water with short breaks between them lead to persisting growth of blood pressure. Further examinations in the laboratory setting that involved CHT have confirmed this fact.
Does adaptation to immersion in cold water change the nature of the cardiovascular response? A 6-week training course implying daily sessions of immersion of face in cold water has shown to somewhat improve peripheral vasospasm, which is reflected in a significantly smaller increase of DBP during CHT. On the contrary, SBP increases significantly during CHT, same as pulse pressure, which indirectly reflects expansion of the left ventricle's stroke volume. Against the background of the developing bradycardia, reaction allows maintaining cerebral blood flow at the required level [19, 22–25]. Thus, these changes are adaptive and protective in nature. Reactivity of the heart's chronotropic function CHT also decreases after adaptation. But in some subjects, both cardiac and vascular reactivity remained high, which may be due to their genetic characteristics, in particular — those of the vascular response effector link. Reflex-driven regulation of tone of vessels in the skin is controlled by the sympathetic part of the autonomic regulation with the help of α1-adrenoreceptors (constrictor function) and β2-adrenoreceptors (dilator function). The degree of reflex response depends on the ratio of these receptors and the effectiveness of their functioning, which are largely determined on the genetic level. However, there is another factor that affects reflex-driven vascular reactions: biochemical background, which depends, in particular, on the activity of the renin-angiotensin and kinin-bradykinin systems. Thus, using CHT, we have shown that in the context of the diving reflex vascular tone and blood pressure significantly depend on the polymorphism of genes encoding ADRA1A (rs1048101), BDKRB2 (rs1799722), ADBR2 (rs1042713), and ACE (I/D, rs4340) [12, 13].
CONCLUSIONS
Disruption of operation of the cardiovascular system pose the greatest risk associated with cold water swimming. It should be remembered that, under the influence of cold water, practically healthy people with adequate body response to physical exertion may have the functions of their cardiovascular systems changing pathologically, with development of cardiac arrhythmias, including fatal ones, blood pressure spikes and the consequences thereof. Body's regulatory systems, autonomic nervous system in particular, can trigger reflex defense mechanisms in specific ways that increase the risk of a pathological response to a cold stimulus. The key factor is the increased reactivity to extreme stimuli of the autonomous regulatory circuit and its effector link, the myocardium, as well as the smooth muscle walls of peripheral vessels of the skin, non-functioning muscles, and the gastrointestinal tract. In this connection, it is necessary to develop additional tests and criteria for winter swimming athletes' training and competition admission examinations. In our opinion, CHT, carried out with ECG recorded and blood pressure controlled, can be very informative for identifying people facing higher risks on the part of the cardiovascular system.