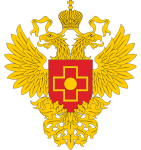
ORIGINAL RESEARCH
Impact of persistent cold stress on spectral characteristics of EEG alpha and theta rhythms in military academy cadets
1 University of Tyumen, Tyumen, Russia
2 Proshlyakov Tyumen Higher Military Engineer Command School, Ministry of Defence of the Russian Federation, Tyumen, Russia
Correspondence should be addressed: Sergey N. Tolstoguzov
Volodarskogo, 6, Tyumen, 625003, Russia; ur.nmtu@vozugotslot.n.s
Author contribution: Tolstoguzov SN — research procedure, data analysis, manuscript writing; Fisher TA — data analysis, manuscript writing; Naida YuV — research procedure; Lepunova ON — data analysis.
Compliance with the ethical standards: the study was approved by the Ethics Commitee of the University of Tyumen (protocol № 11 dated 19 February 2024). All patients submitted the informed consent to stady participation.
Estimation of the effects of cold environment on body’s functional state in humans and assessment of the body’s regulatory system response to cold are the important components of the integrated picture of the stress effects of high-latitude climate on human health, well-being and performance.
The phenomenon of cold exposure training and understanding of physiological mechanisms underlying the human body transition from the not adapted to cold state to the state of stable cold resistance are the important elements of cold stress research. In this regard, it is important to consider the cost of adaptation (allostatic load) paid by humans when adjusting to the acute or prolonged exposure to low temperatures. The studies of thermal homeostasis in both Russian students (young men, who were born and permanently reside in the climate of West Siberia) and foreign students coming from the regions with tropical and subtropical climate are of great interest [1, 2]. Today, geographical migration of the youth from the countries of Africa, Asia, Middle East aimed at getting primary education in the RF shows an upward trend [3]. However, there is almost no description of the types of adaptation (acclimation, acclimatization) to sudden temperature changes associated with the change in geographical conditions. At the same time, there is little research on the body’s functional states and the dynamics of morbidity among foreigners, especially those, who attend military higher education institutions, relative to students living in the RF.
Bioelectric activity of the brain recorded by EEG is a sensitive and, which is more important, informative indicator of body’s adaptive changes and compensatory processes in the central nervous system (CNS) [4–8]. The effects of low temperatures on formation of the brain bioelectric activity, specific EEG patterns in individuals constantly or from time to time exposed to cold are reported in a number of domestic [9–11] and foreign studies [10–12]. Thus, the increase in total spectral power of alpha and theta waves was observed in the EEG patterns of one group of subjects under exposure to cold [7]. The other study, in contrast, reported the cold related decrease in the alpha rhythm power [10]. The literature provides conflicting data on the impact of hypothermia and regular cold exposure training on bioelectric activity of the brain, which are largely dependent on the aspect of the issue considered (acute hypothermia or prolonged exposure, deliberate cold exposure training or inevitable impact of cold high-latitude climate, study of aboriginal or immigrant community, etc.). Furthermore, the issue of the search for EEG markers of successful adjustment of humans to cold exposure is still relevant.
The study was aimed to assess the impact of the military academy cadets’ incremental exposure to the graduated cold stress (cold exposure training) on the EEG spectral characteristics in the alpha and theta ranges.
METHODS
The cross-sectional study involved 58 subjects divided into three groups. The first group of 18 individuals (hereinafter, control group, civil students, CG) consisted of young men studying at the University of Tyumen, who were permanent residents of the Tyumen region; their average age was 21.36 ± 1.83 years. The second group of 29 individuals (hereinafter, experimental group 1 undergoing cold exposure training, military cadets from various regions of the RF, CETG1) consisted of military cadets studying at the Proshlyakov Tyumen Higher Military Engineer Command School, who were permanent residents of the Tyumen region and the regions of the Urals or Siberian Federal District of the RF; their average age was 20.58 ± 1.29 years. The third group of 11 individuals (hereinafter, experimental group 2 undergoing cold exposure training, foreign military cadets, CETG2) consisted of military cadets studying at the Proshlyakov Tyumen Higher Military Engineer Command School, who were permanent residents of the countries with tropical and subtropical climate (Nicaragua, Congo, Mozambique, Guinea, Guinea-Bissau, Gabon) and had dark skin; their average age was 23.39 ± 1.95 years.
Inclusion criteria: health status group 1–2; good academic performance (learning success as a criterion of primary activity efficiency). Exclusion criteria: history of neurological disorder and/or exacerbation of any disease within two weeks before the study.
The study was conducted in mid-November. It was preceded by the 2.5 month training aimed to improve cold resistance in military cadets of both experimental groups in accordance with the program of incremental exposure to the graduated cold stress aimed at improving the servicemen’s adaptive capacity. The program included the entry level training aimed to improve cold resistance (regular dousing with cold (+5…+8 °С) water outdoors, training was conducted 2–3 times a week throughout 3 weeks) [13] and the special training (immersion in cold (+2…+2.5 °С) water in full combat gear, training was conducted 1–2 times a week throughout 2 months) [14].
The stationary Neuron-Spectrum-4/EPM EEG system (Neurosoft; Russia) was used for EEG recording. A total of 16 active electrodes were placed over two hemispheres following the 10–20 system, unipolar configuration with ear references was used. Background EEG was recorded in the relaxed wakefulness states with the eyes closed in the soundproof darkened room. The recording range was 0.5–35 Hz. The electrode resistance was < 20 kOhm. The sampling rate of 500 points was used. The background EEG recording was assessed based on the 20 epochs, 10–15 s each, by selecting the artifact-free segments. The EEG mathematical analysis was performed using the Neuron-Spectrum software for Fourier transform in the θ (4.0–8.0 Hz) and α (8.0–14.0 Hz) frequency ranges. The total spectral power (µV2), rhythm indices (Hz), integrated EEG alpha/theta indices were used to describe the functional state of the subject’s brain.
Statistical data processing was performed using the SPSS Statistics 23 software package. The data were presented as median (Ме), first and third quartiles (Q1–Q3). The distribution was tested for normality using the Shapiro–Wilk test. The distribution of indicators was non-normal, therefore, comparison with controls was performed by nonparametric methods using the Mann–Whitney U test for two independent groups.
RESULTS
Visual assessment of the subjects’ EEG recordings allowed us to distinguish the major variants of the brain bioelectric activity organization in accordance with the classification by E.A. Zhirmunskaya [15] (fig. 1).
In the control group, type I bioelectric activity (66% of cases) showing the organized alpha activity structure and the pronounced anterior-posterior gradient of the background rhythm prevailed. Type II and III EEG patterns were less frequent: 28 and 6% of cases, respectively. In the experimental groups 1 and 2, which were through cold exposure training, a hypersynchronous EEG type showing the evident alpha rhythm with no spindle events was the most common (55 and 64%, respectively). In 20% of cases, young men in the experimental group 1, who underwent cold exposure training, also showed a type III asynchronous EEG pattern with the sharp decrease in alpha rhythm and substitution of alpha rhythm with oscillations in the theta and beta ranges.
The EEG spectral power analysis in the theta range revealed significantly higher values in the right hemispheric leads in the experimental group 2 undergoing cold exposure training compared to controls (fig. 2).
Thus, the subjects in CETG2 were significantly superior to the CG in theta activity recorded by the following electrodes: right anterior frontal Fp2 (U = 26; Z = –3.28; p < 0.001), right frontal F4 (U = 29; Z = –3.14; p = 0.002), right central С4 (U = 31; Z = –3.05; p = 0.002), right anterior temporal F8 (U = 49; Z = –2.24; p = 0.024), right temporal T4 (U = 10; Z = –4.00; p < 0.001), right parietal Р4 (U = 24; Z = –3.37; p = 0.001), and right occipital О2 (U = 45; Z = –2.42; p = 0.015). Young men in CETG1, on the contrary, had significantly lower total spectral power values in theta range compared to controls based on the activity recorded by the following electrodes: left and right CETG2 — experimental group 2 undergoing cold exposure training anterior frontal Fp1 (U = 119.5; Z = –3.09; p = 0.002), Fp2 (U = 120; Z = –3.07; p = 0.002), left central С3 (U = 165; Z = –2.10; p = 0.035), right anterior temporal F8 (U = 161; Z = –2.17; p = 0.029), left and right temporal T3 (U = 142; Z = –2.60; p = 0.009), T4 (U = 151; Z = –2.39; p = 0.016), left and right posterior temporal T5 (U = 112; Z = –3.26; p = 0.001), T6 (U = 173; Z = –1.92; p = 0.050).
In the alpha band, the total spectral power was also higher in CETG2, it was significantly superior to that of CG in the following leads: right anterior frontal Fp2 (U = 23; Z = –3.41; p < 0.001), right frontal F4 (U = 24; Z = –3.37; p < 0.001), right central С4 (U = 46; Z = –2.38; p = 0.017), right anterior temporal F8 (U = 33; Z = –2.96; p = 0.003), left and right temporal T3 (U = 54; Z = –1.95; p = 0.050), T4 (U = 13; Z = –3.86; p < 0.001) (fig. 3).
The total spectral power in the alpha range of the CETG1 subjects was significantly lower compared to that of controls based on the activity recorded by the following electrodes: left temporal T3 (U = 170; Z = –1.98; p = 0.042) and left posterior temporal T5 (U = 171; Z = –1.92; p = 0.050).
The theta index of the experimental group 2 undergoing cold exposure training exceeded the values of the control group over the almost entire convex surface, except for С3, F8 and T5, based on the activity recorded by the following electrodes: left and right anterior frontal Fp1 (U = 28; Z = –3.19; p = 0.001), Fp2 (U = 7; Z = –4,13; p < 0,001), left and right frontal F3 (U = 53; Z = –2.06; p = 0.038), F4 (U = 44; Z = –2.47; p = 0.013), right central С4 (U = 20; Z = –3.55; p < 0.001), right anterior temporal F8 (U = 39.5; Z = –2.67; p = 0.007), left and right temporal T3 (U = 42; Z = –2.56; p = 0.010), T4 (U = 8; Z = –4.09; p > 0.001), right posterior temporal T6 (U = 26.5; Z = –3.25; p = 0.001), left and right parietal Р3 (U = 3; Z = –4.31; p < 0.001), Р4 (U = 0; Z = –4.44; p < 0.001), left and right occipital О1 (U = 45; Z = –2.42; p = 0.015), О2 (U = 0; Z = –4.44; p < 0.001) (tab. 1).
The extent of theta oscillations in the experimental group 1 undergoing cold exposure training was the same as in the control group.
The EEG alpha index of the CETG2 subjects exceeded that of controls in frontal regions of the brain based on the activity recorded by the following electrodes: left and right anterior frontal Fp1 (U = 50; Z = –2.17; p = 0.029), Fp2 (U = 35; Z = –2.87; p = 0.004), right anterior temporal F8 (U = 41; Z = –2.58; p = 0.009). Furthermore, the alpha rhythm index of the CETG2 subjects was significantly lower than that of controls in the midline and caudal regions based on the signals in the following leads: left central С3 (U = 51.5; Z = 2.13; p = 0.033), left and right parietal Р3 (U = 47; Z = 2.33; p = 0.019), Р4 (U = 13; Z = 3.86; p < 0.001), and right occipital О2 (U = 45; Z = 2.43; p = 0.015). In general, the alpha index of the control group 2 undergoing cold exposure training showed no frontal–occipital gradient.
The alpha index of the experimental group 1 undergoing cold exposure training had the zonal specifics and increased in the direction from frontal to caudal regions, the same as in the control group. In the CETG1 subjects, the alpha rhythm index values exceeded that of controls only in the left anterior frontal Fp1 (U = 166; Z = 2.07; p = 0.037) and the right frontal F8 (U = 162; Z = 2.02; p = 0.042) leads.
Then we calculated the index of the cortical-subcortical neurodynamic processes’ stability as an integrated ratio of alpha/theta rhythms based on the total spectral power and rhythm index (tab. 2).
The highest values of the CNS functional state stability were obtained in the experimental group 1 undergoing cold exposure training, while the lowest values that were significantly different from that of the control group were reported in young men in the experimental group 2, who were through cold exposure training.
DISCUSSION
Despite certain conventionality of the classification reported in the number of studies, visual assessment of EEG makes it possible to obtain a comprehensive picture of the formation of the subjects’ brain bioelectrical activity and the brain functional state [16, 17].
Our findings showed that type I EEG with the evident background brain rhythm, it’s clear zonal gradation by index and power modulated into spindles prevailed in the control group consisting of civil young men. It can be assumed that the optimal functional state of the CNS associated with the balanced interaction between the activating mesencephalic and synchronizing diencephalic brain structures was observed in the majority of the control group members. The type II hypersynchronous (monorhythmic) EEG was more often found in two experimental groups of military cadets, which suggested some strain of adjustment mechanisms, rhythmic disorganization, and redistribution of the limbic-diencephalic, thalamocortical and intracortical interactions in the subjects’ brain. The increase in the share of individuals with monorhythmic EEG in our experimental samples and the fact of detecting type III asynchronous (flat) EEG pattern in CETG1 could result from the cadets’ (both Russian and foreign) body incomplete adaptation to the regular cold exposure training conducted as part of the special training program.
The researchers have noted that the CNS plays a key role in the process of cold adaptation and cold exposure training, which is reflected in the brain biorhythm pattern [1]. The other paper also reports that specific EEG patterns that are considered as adaptive can develop in humans due to prolonged cold exposure (as well as due to acute cold exposure) [7].
A number of studies demonstrate high sensitivity of the EEG spectral power in theta and alpha ranges to the stress exposures experienced by the body, which are essentially the markers of successful adaptive responses to various adverse environmental factors [18–20].
In our study, foreign military cadets demonstrated significantly higher total spectral power in the theta and alpha bands in the right hemisphere compared to controls, which could be indicative of the increased autonomic nervous system sympathetic division activation. It is well known that the right hemisphere modulates the sympathetic tone [7], while the autonomic nervous system integrates the functions of internal organs via activation of autonomic centers in the brain [21]. At the same time, the increase in the theta rhythm total power reflects the decrease in the cortical centers’ inhibitory control over the brainstem and subcortical structures [22], and communication between the autonomic nervous system and the overlying nervous centers plays a vital role in developing an adaptive response to cold exposure during the cold exposure training [23].
The alpha rhythm abundance (index) and frontal asymmetry represent an important indicator of stress exposure [24]. In our study, alpha rhythm showed significant asymmetry of the total spectral power and rhythm index values in the right frontal and anterior temporal leads in the group of foreign military cadets (CETG2). It is believed that the emergence of the alpha oscillation frontal asymmetry pattern with increasing bioelectric activity of the right hemisphere is associated with the avoidance system responses and the aggressive-defensive behavioral stereotypes, while alpha activation of the left hemisphere reflects the approach system activity and the orientativeexploratory behavior [25].
There are some research and theoretical papers that consider not the EEG spectral characteristics themselves, but their relationships defined as rhythm indices, as biomarkers of stress [26, 27]. Interaction of the brain bioelectric activity main spectral components can be used as a marker of the homeostatic and adaptation mechanisms’ efficiency [28, 29]. One of such indices, characterizing the adaptation processes and maladaptive disorders, represents the ratio of the alpha/ theta oscillation spectral characteristics.
In our groups of subjects, a functional “α-core” was formed in the samples of civil students and Russian military cadets based in the alpha/theta index, while foreign military cadets showed signs of the α-core disruption by other EEG spectral components, specifically the theta range. Such pattern could be indicative of destabilization of the neurodynamic processes in the CNS associated with the cerebral homeostasis disturbance and incomplete adaptive adjustment of the foreign cadets’ bodies under the influence of the extreme cold weather training programs. Subdominance of alpha and theta components in CETG2 resulted in the formation of binary α-θ structure of brain biorhythms typical for maladaptation with the following further scenarios: transition to the adjusted and cold resistant (weathered) state and formation of α-core on the EEG or transition from early-stage maladaptation to persistent disorder [30].
CONCLUSIONS
The combination of EEG spectral characteristics in alpha and theta ranges, as well as their interaction during formation of the brain background bioelectric activity α-core suggest that the special program involving incremental exposure of military academy cadets to the graduated thermal stress ensured the desired weathering effect in young Russians, which affected the cerebral homeostasis stability with some instability of neurodynamic processes in the CNS. In foreign military cadets, regular cold exposure training resulted in the pronounced disintegration of cortical-subcortical and intracortical interactions, as well as in the formation of binary α-θ structure of background EEG, suggesting incomplete adaptation processes and high stress load on the body.
We have prepared some recommendations based on the findings. First, it is reasonable to extend the entry level cold resistance training of foreign military cadets from 3 weeks to 3–6 months in order to ensure milder adaptation to the graduated short-term cold stress (extend the acclimation period). Second, the findings should be compared with the EEG data of foreign military cadets not engaged in cold exposure training, who are in the same situation of adaptation to the climatic and geographical conditions of West Siberia, in further studies.