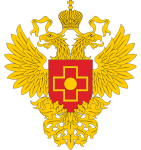
ORIGINAL RESEARCH
Comparison of methods for purification of bacteriophage lysates of gram-negative bacteria for personalized therapy
1 Federal Research and Clinical Center of Physical-Chemical Medicine of Federal Medical Biological Agency, Moscow, Russia
2 Federal Research Center of Biotechnology, Moscow, Russia
Correspondence should be addressed: Roman B. Gorodnichev
Malaya Pirogovskaya, 1a, Moscow, 119435; moc.liamg@b.r.vehcindorog
Funding: all study expenses were covered by the funds allocated for the State Assignment on the Development of a personalized approach to the therapy of infections using virulent bacteriophages (Code: Bacteriophage).
Author contribution: Gorodnichev RB — planned the study, conducted the experiments, and wrote the manuscript; Kornienko MA, Letarov AV, Shitikov EA — planned the study, analyzed its results, and wrote the manuscript; Kuptsov NS, Efimov AD, Bogdan VI — conducted the experiments; Ilina EN — planned the study and wrote the manuscript.
Compliance with ethical standards: the experiments were conducted in full compliance with Biosafety Guidelines for working with risk group III–IV pathogens (SP 1.3.2322-08), Amendment 1 to Biosafety Guidelines for working with risk group III–IV pathogens (SP 1.3.2518-09), medical waste regulations (SanPin 2.1.7.279010), and Federal Clinical Guidelines on the rational use of bacteriophages for therapy and prevention of diseases.
Irrational use of antibiotics has driven the emergence and global dissemination of drug-resistant microorganisms. The World Health Organization has prioritized the search for novel antimicrobial agents against Acinetobacter baumannii, Pseudomonas aeruginosa, Klebsiella spp, Escherichia coli, and Enterobacter spp. because the number of multidrug-resistant isolates in this group of microorganisms has been steadily trending upwards [1].
As alternatives to antibiotics, virulent bacteriophages hold promise for the therapy of multidrug-resistant bacterial infections [2]. Phages are naturally occurring antagonists of bacteria, capable of selectively and effectively infecting a bacterial cell. The primary advantage of phage therapy lies in the ability of phages to cause death of the bacterial cell regardless of its sensitivity to antibiotics [3]. Besides, due to remarkable host specificity, phage therapy can eliminate the infectious agent without harming the patient’s natural microbiota.
Based on the accumulated clinical experience, the following requirements have been elaborated for therapeutic phages and phage cocktails: phage virulence, the absence of toxin genes and antibiotic resistance determinants in the phage genome, the ability to infect a wide range of hosts, which is determined by the efficiency of plating, high levels of phage particle production per cell, and the effective phage concentration of at least 109 PFU/ml [4–7].
However, as typical viruses phages replicate only inside a bacterial cell, usually causing its lysis. So, phage preparations can be contaminated by bacterial cells and their components, including endotoxins [6]. Lipopolysaccharides, which are the main constituent of the outer membrane of gramnegative bacteria, are the most illustrative example of bacterial endotoxins [8]. Endotoxins are highly immunogenic and can provoke septic (endotoxic) shock resulting in intravascular coagulation, organ failure and death [9].
So far, a few approaches to phage cultivation and purification have been proposed; of them, polyethylene glycol (PEG) precipitation and CsCl density gradient ultracentrifugation are the most common [10]. The drawback of these approaches is the presence of harmful compounds (e.g. cesium salts (80 ng/ml) in the end preparation with CsCl centrifugation), which significantly limits its usage as a therapeutic agent [10]. Sucrose density gradient ultracentrifugation exploited by virology studies may offer an alternative [11, 12]. Methods of phage enrichment from environmental sources, too, have been increasingly used for the purification of phage lysates in recent years; among them are 100 kDa filtration and isolation in the presence of salts [10, 12–14]. Several chromatography-based methods for phage purification have been developed, like affinity chromatographybased commercial products for removing endotoxins from phage lysates, for example, EndoTrap® columns (Lionex GmbH; Germany). However, a series of research studies have shown that a single passage of a crude lysate through the column only slightly reduces endotoxin concentrations in the preparation [13, 15, 16]. The aim of this study was to compare the efficacy of purification methods for phage lysates intended for therapeutic use.
METHODS
Bacterial strains, phages, and media
The K. pneumoniae strain KP9068 was obtained from the collection of the Laboratory of Molecular Genetics of Microorganisms (Federal Research and Clinical Center of Physical-Chemical Medicine, FMBA; Russia). This strain is resistant to cephalosporins (cefotaxime, ceftazidime, ceftriaxone, cefixime), fluoroquinolones (ciprofloxacin, ofloxacin), tetracycline, azithromycin, chloramphenicol, gentamicin, and ampicillin and has intermediate sensitivity to meropenem. The strain belongs to sequence type 11. Its capsule type identified by the sequencing of the wzi gene is K23 [17]. Bacterial cultures used in our experiment were grown in lysogeny broth (LB, Himedia; India) at 37 °С.
Drawing on the literature data on the phylogenetic diversity of bacteriophages in commercial phage cocktails, we picked 2 lytic phages that represented 2 different families and were active against K. pneumoniae strains with K23 capsule type [18].
Bacteriophages vB_KpnM_Seu621 (MT939253.1) and vB_KpnP_Dlv622 (MT939252.1) cause lysis of K. pneumoniae and belong to the Myoviridae and Autographiviridae families, respectively [19]. Phage vB_KpnM_Seu621 has the following morphological characteristics: an isometric head of 75 nm in diameter and a 104 nm long contractile tail. Bacteriophage vB_KpnP_Dlv622 has a smaller isometric head (57 nm) and a 12 nm long non-contractile tail. The genomes of the 2 bacteriophages were analyzed in earlier work and did not encode any toxin and integrase genes that could preclude their use in therapy [19].
The phages were grown using the KP9068 strain of K. pneumoniae as a host in a thermoshaker at 100 rpm and 37 °C for 18 h. Briefly, the host strain was grown to the midlog phase (OD 600 nm = 0.3) and then inoculated with the phage lysate to achieve the multiplicity of infection of 0.001. The obtained phage lysates were clarified from cell debris by 15 min centrifugation at 3,500 g followed by sequential filtering through 0.45 µm (Merck Millipore; USA) and 0.22 µm (Merck Millipore; USA) membrane filters. Phage concentrations in the phage lysate were measured using the standard double-layer agar technique. Phage titers were expressed as the number of plaque forming units per one ml (PFU/ml) [20].
Phage precipitation with PEG
In this step, phage particles were recovered from the lysate by precipitation with PEG 6000 (Dia-M; Russia) using a previously described but slightly modified technique [21]. Ten ml of the phage lysate was combined with 2.5 ml of a sterile solution containing 20% PEG 6000 and 2.5 M NaCl. The samples were mixed by inverting without vortexing, cooled on ice for 1 h and centrifuged at 20,000 g for 20 min. Most of the supernatant was carefully removed. Then the samples were centrifuged again at 20,000 g for 10 min and the supernatant was again removed. The precipitate containing phage particles was resuspended in 1 ml of a sterile SM buffer (100 mM NaCl, 10 mM MgSO4, 50 mM Tris-HCl, pH 7.5, and 0.01% (w/v) gelatin. The suspension was vortexed, incubated on ice for 1 h, vortexed again to remove PEG, and centrifuged at 13,000 g for 1 min. The supernatant was transferred to a clean testing tube for further experiments.
CsCl density gradient ultracentrifugation
CsCl density gradient ultracentrifugation was performed following the protocol described in [21]. Aliquots (70 ml) of the phage lysates were centrifuged in a Beckman Type 45Ti rotor (Beckman Coulter; USA) at 75,000 g and 20 oC for 1 h. The supernatant was gently removed and the precipitate was resuspended in 800 µl of SM buffer. Next, solutions containing different concentrations of CsCl (1.3–1.4–1.45–1.5–1.6 g/ml) and SM buffer were prepared. The solutions (800 µl) were added to the centrifuge tube layer by layer to create a gradient, starting with the highest CsCl concentration; the top layer solution had the lowest CsCl concentration. A concentrated phage sample (800 µl) containing only one of the studied phages was applied on top of the last layer of the gradient. The samples were centrifuged in a Beckman SW50.1 rotor (Beckman Coulter; USA) at 75,000 g and 20 оС for 1 h. The opalescent phage band was collected, brought to 1 ml by adding SM buffer, and placed into a dialysis tubing (Thermo FS; USA). Dialysis was performed for 18 h against a tenfold volume of SM buffer at 4 °C and constant stirring of the liquid, replacing the buffer every 4 h. After dialysis, the samples were additionally filtered through 0.22 µl filters (Merck Millipore; USA).
Sucrose density gradient ultracentrifugation
Sucrose density gradient ultracentrifugation was performed following a standard but slightly modified protocol [11].
Aliquots (70 ml) of the phage lysates were centrifuged in a Beckman Type 45Ti rotor (Beckman Coulter; USA) at 75,000 g and 20 °C for 1 h. Each of the obtained precipitates was resuspended in 800 µl of SM-buffer. Then sucrose solutions (20, 30, 40, 50 and 60%) were prepared in a buffer containing 50 mM Tris-НCl, 50 mM NaCl, pH 7.5. The solutions (800 µl) were added to the centrifuge tube layer by layer to create a gradient, starting with the solution that had the highest sucrose concentration (60%); the top layer solution had the lowest sucrose concentration (20%). A concentrated phage sample (800 µl) containing only one of the studied phages was applied on top of the last layer of the gradient. The samples were centrifuged in a Beckman Type SW50.1 rotor (Beckman Coulter; USA) at 75,000 g and 20 °C for 1 h. Free phage particles formed a visible opalescent band between the 50% and 60% sucrose layers. The phage layer was collected and brought to 1 ml by adding SM buffer.
Phage purification with 100 kDA centrifugal filters
The phage lysates (1 ml) were placed on 100 kDa Microcon centrifugal filters (Millipore; USA) and centrifuged at 10,000 g for 10 min. To recover trapped phage particles, the filter was coated with 300 µl of sterile SM buffer, placed upside down in a clean sterile centrifuge tube and centrifuged at 1,000 g for 3 min. The purified lysate was brought to 1 ml by adding a sterile SM buffer.
Phage concentration on 0.22 µm cellulose filters in the presence of MgSO4
Phage concentration on 0.22 µm filters in the presence of MgSO4 was performed on 50 ml aliquots of the lysates cleared of cell debris [14]. Dry reagent-grade MgSO4 (Dia-M; Russia) was added to the lysates to reach a final concentration of 50 mM. The samples were mixed by inverting without vortexing until complete dissolution of MgSO4. The obtained suspensions were slowly filtered through 0.22 µm mixed cellulose ester GSWP membranes (Millipore; USA). The membranes were cut in small pieces (sized ~ 1 mm2) and immersed into 5 ml of a sterile SM buffer. To achieve complete elution of phage particles from the filter surface, the suspension was ultrasonicated treated by ultrasonication for 4 min. Then, large filter fragments were precipitated by 10 min centrifugation at 3,500 g; the supernatant was collected for further experiments.
Measuring endotoxin concentrations in the samples
Endotoxin concentrations were measured using commercial Endosafe® kits for turbidimetric LAL testing (Charles River; USA) following the manufacturer’s protocol. Turbidity assays were conducted in a microplate reader (Multiskan Ascent, Thermo; USA) at 405 nm wavelength.
Statistical analysis
Statistical analysis (ANOVA, standard deviations) was carried out in GraphPad Prisma v.8.0.1 (GraphPad Software; USA).
RESULTS
Study design
Five different methods of phage purification were compared: precipitation with PEG, CsCl density gradient ultracentrifugation, sucrose density gradient ultracentrifugation, purification with 100 kDA centrifugal filters, and concentration on 0.22 µm cellulose filters in the presence of MgSO4. Each of the studied purification procedures was conducted in 3 replicates. Their efficacy was assessed by measuring phage titers and endotoxin concentrations in the purified product.
First, phage lysates were obtained with the following phage titers: 1.25 × 1012 ± 7.46 × 1010 PFU/ml and 2.25 × 1012 ± 1.34 × 1011 PFU/ml for vB_KpnM_Seu621 and vB_KpnP_Dlv622, respectively. Bacterial debris and live bacterial cells were removed by filtration through 0.22 µm filters (Millipore; USA). Endotoxin concentrations in the non-purified phage lysates were 3,806,056 ± 429,410 EU/ml for vB_KpnM_Seu621 and 189,456 ± 12,406 EU/ml for B_KpnP_Dlv622 (fig. 1).
The obtained phage preparations were tested for contamination by seeding their 50 µl aliquots on nutrient agar. Mean values and standard deviations were calculated for the measured phage titers and endotoxin concentrations. We also calculated endotoxin concentrations in a single therapeutic dose of the phage preparation. Drawing on the literature, we assumed the effective therapeutic phage dose to be 109 [22–24].
Changes in endotoxin concentrations after applying different purification methods
In the first part of our study, we analyzed the efficacy of conventional laboratory methods for phage purification: precipitation with PEG and CsCl density gradient ultracentrifugation. Phage precipitation with PEG reduced
endotoxin concentrations by 2–3 orders of magnitude (p < 0.0001): from 3,000 ± 324 to 3,817 ± 486 EU/ml. CsCl density gradient ultracentrifugation is the gold standard of phage purification; it resulted in an even greater reduction of endotoxin concentrations: from 303 ± 35 to 313 ± 20 EU/ml (p < 0.0001) (see fig. 1).
Sucrose density gradient ultracentrifugation, 100 kDa filtration and slow filtration through 0.22 µm cellulose filters in the presence of MgSO4 were chosen as alternative purification methods that could produce safe therapeutic formulations suitable for clinical use. Sucrose density gradient ultracentrifugation reduced endotoxin concentrations in the vB_KpnM_Seu621 lysate by 4 orders of magnitude (p < 0.0001), to 200 ± 28 EU/ml; for the vB_KpnP_Dlv622 lysate, the reduction was not so pronounced: by only 2 orders of magnitude, to 3,368 ± 348 EU/ml (p < 0.0001). Using 100 kDa centrifugal filter concentrators, we were able to lower endotoxin concentration by 1.5 orders of magnitude (p < 0.0001) in both lysates, bringing them down to 56,148 ± 7,832 EU/ml for phage vB_KpnM_Seu621 and 3,850 ± 593 EU/ml for phage B_KpnP_Dlv622. The efficacy of phage isolation in the presence of MgSO4 was similar to that of PEG precipitation (p > 0.9999) and resulted in a reduction in endotoxin concentrations by 2–3 orders of magnitude: from 3,187 ± 368 to 3,502 ± 372 EU/ml (p < 0.0001) (see fig. 1).
Changes in phage titers after applying different purification methods
A phage titer is an important characteristic of a therapeutic phage formulation; therefore, it needs to be either maintained or increased through purification.
Different purification methods produced different changes in phage titers. Precipitation with PEG did not change vB_KpnM_Seu621 titers significantly. By contrast, vB_KpnP_ Dlv622 titers dropped by 3 orders of magnitude (p < 0.0001) to 1 × 109 ± 5.7 × 107 PFU/ml after PEG precipitation (fig. 2). The gold standard of purification, CsCl density gradient ultracentrifugation, demonstrated a more uniform result: in both phage preparations, phage titers did not change significantly (p > 0.9999) (fig. 2).
Sucrose density gradient ultracentrifugation led to a reduction (p < 0.0001) in vB_KpnM_Seu621 titers to 5 × 109 ± 2.9 × 108 PFU/ml but did not change vB_KpnP_Dlv622 titers significantly. Filtration with 100 kDa centrifugal filters reduced (p < 0.0001) phage titers in both phage preparations down to 1.5–5 × 1010 ± 3.1 × 109 PFU/ml. Filtration in the presence of MgSO4 reduced (p < 0.0001) phage titers by 2–3 orders of magnitude, to 1.5 × 1010 ± 1.1 × 109 PFU/ml and 5 × 109 ± 2.5 × 108 PFU/ml for vB_KpnM_Seu621 and vB_KpnP_Dlv622, respectively (see fig. 2).
DISCUSSION
Phage therapy has been used in clinical practice since the beginning of the 20th century. It is effective against infectious diseases of any etiology, especially against purulent and inflammatory wounds, otitis and bowel infections [25–27]. As more bacteria are acquiring multidrug resistance, the need arises to administer phage preparations intravenously or intrathecally. Phage formulations for intravenous administration must satisfy certain purity and efficacy criteria. According to the pharmacopeial description provided in the Pharmacopoeia Monograph.1.2.4.0006.15 on Bacterial endotoxins, the amount of endotoxin in the formulation cannot exceed 5 EU/kg per hour for an intravenous formulation and 0.2 EU/kg per hour for an intrathecal formulation [28] According to the literature, the recommended phage titer ensuring a stable bactericidal effect is at least 109 PFU per dose [22–24].
For the purpose of our study, we selected 2 phages from two different families. Both phages vB_KpnM_Seu621 and vB_KpnP_Dlv622 caused lysis of the bacterial host, and the concentrations of phage particles in the lysates were very similar (1.25–2.25 × 1012 ± 1.34 × 1011 PFU/ml), but the detected amount of endotoxin in the phage lysates differed twentyfold (see fig. 1). This finding is consistent with the literature reports: endotoxin concentrations in phage lysates vary considerably (101–105 EU/109 PFU) depending on the taxonomic position of the phage [10, 12, 13]. Based on the standard guidelines on the minimum phage titer in the therapeutic dose (109 PFU/ml) and the maximum allowed endotoxin concentration (325 EU/ml) in an intravenous phage formulation (calculated for a single 1 ml dose administered intravenously to a patient weighing 65 kg), we conclude that the lysate of phage vB_KpnP_Dlv622 can be used for intravenous administration after dilution, unlike the lysate of phage vB_KpnM_Seu621 with endotoxin concentrations being by an order of magnitude higher than the admissible maximum level (fig. 1).
Being the gold standard of phage purification, CsCl density gradient ultracentrifugation turned out to be most effective in comparison with other tested methods (see fig. 1). There are a few reports of successful phage therapy with intravenous phage formulations purified with CsCl density gradient ultracentrifugation [22, 23]. However, this purification method has its own limitations due to the use of cesium salts because the end preparation, even when dialyzed, may contain residual cesium [10]. According to the literature, CsCl density gradient ultracentrifugation can remove up to 99.6% of the total endotoxin amount, but generally its efficacy varies from 18% to 99.6% [13].
Phage precipitation with PEG is a common laboratory method for phage purification. In our study, its efficacy differed between phage families. After PEG purification, the preparation of vB_KpnM_Seu621, the representative of the Myoviridae family, still contained a fairly high vB_KpnM_Seu621 titer. But the titer of phage vB_KpnP_Dlv622 fell significantly after this procedure, showing its low efficacy for the Autographiviridae family. According to the literature, PEG precipitation is not equally effective for morphologically different phages, and it may be necessary to adjust the composition of the salt fraction or the length of the PEG molecule [10, 12, 29]. PEG precipitation removes up to 88% of endotoxin from the lysate of the filamentous E. coli phage М13 and reduces the amount of endotoxin twentyfold for the Myoviridae family [12].
Sucrose density gradient ultracentrifugation is widely used for the purification of virus suspension; however, it is rarely exploited to purify phage lysates [11]. In our study, this method generated purified phage preparations suitable for intravenous and even intrathecal administration, as was the case with vB_KpnP_Dlv622. It should be noted that due to the reduction in phage titers, we were able to obtain only 7 doses of vB_KpnM_Seu621 preparations, which may not be sufficient for one complete course of personalized therapy. The problem could be solved by optimizing the protocol by adjusting the concentration of the gradient solution and centrifugation time.
An endotoxin molecule is usually no larger than 10–20 kDa, so 100 kDa filtration may be effective for phage purification. In our study, the efficacy of this method differed between the phages and caused a reduction in phage titers in both preparations. For the Myoviridae phage, high endotoxin concentrations might be explained by the presence of endotoxin micelles that grow to 1,000 kDa and therefore cannot pass the filter [30]. Earlier, tangential flow filtration, a modified version of 100 kDa filtration, was successfully used to effectively eliminate up to 90% of endotoxin from the phage lysate; however, this method was never tested on Podoviridae phages [10].
Slow filtration through 0.22 µm cellulose filters in the presence of MgSO4 is normally used for phage enrichment from natural water sources but can be applied to purify phage lysates, too. Despite the fall in phage titers during purification, this method generated a sufficient number of therapeutic doses suitable for intravenous administration. Importantly, the efficacy of the method was satisfactory for both phages regardless of their taxonomic position and thus was the only alternative for the purification of Myoviridae phages.
Slow filtration in the presence of MgSO4 and 100 kDa filtration turned out to be the least time-consuming, taking 2 and 1 h, respectively. Sucrose density gradient centrifugation and PEG precipitation took an average of 3.5 h. However, phage lysates purified with CsCl density gradient centrifugation and precipitation with PEG required further purification with dialysis, which extended purification time to 18 h. So, the most rapid methods for producing purified phage preparations suitable for clinical use were 100 kDa filtration, slow filtration in the presence of MgSO4, and sucrose density gradient ultracentrifugation.
Our findings were consistent with the literature: the amount of endotoxin after purification and the efficacy of the tested purification methods were different for different phage families. Due to the difference in phage size and/or the value of the sedimentation constant, an optimal purification method or a combination of purification methods will depend on the phylogenetic position of the bacteriophage.
CONCLUSIONS
Phage purification methods or their combination should be selected individually depending on the characteristics of a given phage. Nevertheless, sucrose density gradient centrifugation and slow filtration in the presence of MgSO4 could be considered as the most promising for the purification of Myoviridae and Autographiviridae phage lysates. These methods yield safe sufficiently purified phage preparations containing permissible amounts of endotoxins.