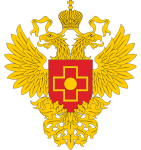
ORIGINAL RESEARCH
Assessment of individual hematopoietic stem cell response to gamma exposure using humanized mice
1 Urals Research Center for Radiation Medicine of the Federal Medical Biological Agency, Chelyabinsk, Russia
2 Chelyabinsk State University, Chelyabinsk, Russia
Correspondence should be addressed: Natalia I. Atamanyuk
Vorovskogo, 68А, Chelyabinsk, 454141, Russia; ur.liam@arhlup_ativ
Funding: the study was performed as part of the State Assignment of FMBA of Russia.
Acknowledgments: the authors would like to thank A.V. Sherstobitov (Regional Perinatal Center, Chelyabinsk) for his help in organizing and cord blood sampling.
Author contribution: Atamanyuk NI — planning and conducting experiments, manuscript writing; Pryakhin EA — study planning and management, manuscript writing; Styazhkina EV — HSC isolation, data analysis; Obvintseva NA — measurement, flow cytometry; Tryapitsyna GA — data analysis; Peretykin AA — animal exposure, dosimetry studies; Andreev SS — animal handling, measurement; Aldibekova AE — animal handling; Akleyev AV — general management.
Compliance with the ethical standards: the study was approved by the Ethics Committee of the Urals Research Center for Radiation Medicine (protocol № 2 of 27 June 2022). Cord blood sampling was performed after obtaining the informed consent from donors at the Chelyabinsk Regional Perinatal Center. Peripheral blood samples were provided by the Blood Transfusion Station of FMBA of Russia (Chelyabinsk) in accordance with the Decree of the Government of the Russian Federation № 331 of 12 April 2013. Animals were handled in accordance with the European Convention for the Protection of Vertebrate Animals used for Experimental and other Scientific Purposes (1986, Strasbourg), Directive 2010/63/EU of the European Parliament and of the Council of 22 September 2010 on the protection of animals used for scientific purposes.
Developing the approaches to estimation of individual radiosensitivity remains a priority of human radiation biology due to rapid development of nuclear technologies, nuclear medicine technologies and the perspectives of long-term interplanetary spaceflights [1, 2]. Assessment of individual response to exposure is necessary to identify the groups with high risk of exposure effects. This would be useful for both selection of staff to work with the sources of ionizing radiation and cosmonauts, and personalization of the risk of health effects of human exposure to radiation.
The phenomenon of radiosensitivity is assessed by the researchers at various levels, from radiosensitivity of the whole body manifested in survival or death after exposure, to radiosensitivity of tissues and cells and their susceptibility to the long-term adverse exposure effects [1–3]. However, there is no uniform system of determining individual radiosensitivity in humans. Intensive research focused on studying molecular genetic, immunological, hematological and other markers, the personalized predictors of the tissue response and the longterm effects of both acute and chronic exposure to radiation, is under way [1, 2, 4, 5].
Red bone marrow is one of the most radiosensitive tissues in the human body. The red bone marrow resistance to radiation exposure depends on the DNA damage repair, cell repopulation in the tissue on the accounts of proliferating revenant cells, the ability of the tissue to form the functional reserve of cells, etc. [1]. In the bone marrow form of acute radiation syndrome, the effects on the body (the chance of death) result largely from the hematopoietic stem cell (HSC) survival rate and the kinetics of the revenant cell populations [6, 7]. Ionizing radiation has a suppressive effect on the HSC proliferative and regenerative potential. Furthermore, the issue of the relationship between the HSC response to exposure and individual radiosensitivity remains unresolved.
The use of humanized mice for assessment of individual human HSC response to ionizing radiation is promising [8, 9]. The following types of response to radiation exposure have been reported in the model mice humanized by transplantation of human HSCs: enhanced γH2AX foci, increased expression of the p16INK4a gene, loss of HSC capability of repopulation after transplantation to secondary recipients, and reduced differentiation repertoir [10]. Similar processes that are typical for the HSC natural ageing [11–13] are also observed in cases of human or animal external exposure [14]. In particular, clonal expansion of hematopoietic cells was reported in exposed animals [15] and astronauts who experienced high levels of radiation exposure during spaceflights [16].
The above defines the relevance and validity of such task as the development of methods for estimation of the HSC individual response to exposure and its relation to radiosensitivity.
The study was aimed to develop the technology for estimation of the human HSC individual response to exposure based on the HSC xenotransplantation to immunodeficient mice in order to determine radiosensitivity in terms of the hematopoietic tissue response to acute radiation exposure.
METHODS
The NOD SCID immunodeficient mice (breeding nursery of the SPF vivarium, Institute of Cytology and Genetics, Siberian Branch of Russian Academy of Sciences; Novosibirsk, Russia) were used to obtain humanized mice. Animals were housed in the specific pathogen free (SPF) environment with a temperature of 22 ± 2 °С and humidity of 50–60% and fed with the autoclaved SPF pellets for stock mice. They were given free access to food and water, the 12-hour light cycle was used. Cervical dislocation was used to withdraw animals from the experiment.
Animals were administered peripheral blood and cord blood HSCs [17]. HSCs were obtained from the cord blood samples collected in the Regional Perinatal Center and from the donor peripheral blood product, the buffy coat (Blood Transfusion Station of FMBA of Russia; Chelyabinsk, Russia). HSCs were isolated from blood by innumomagnetic separation using the EasySep Human Cord Blood CD34 Positive Selection Kit II (Stem Cell Technologies; Canada). HSCs were identified as the CD45lowCD34+ cells.
The mice received intravenous lateral tail vein injections of the cord blood HSCs after acute exposure to the external gamma dose of 2.5 Gy. The HSC engraftment in the murine bone marrow and the development of human lymphogranulopoiesis occurred within nine weeks. Equal amounts of HSCs collected from each cord blood donor were administered to at least three mice (the number of administered HSCs was 30,000– 200,000 cells per animal). One mouse was left as a control, while the other two were exposed to the doses of 0.5, 1.0, and 1.5 Gy nine weeks after the HSC transplantation (three HSC donors per dose). The number of human and murine cells was measured in the femurs of the control mouse and other mice three days and 14 days after exposure.
HSCs isolated from peripheral blood were administered to mice by intraosseous tibia injection after applying isoflurane anesthesia. Equal amounts of HSCs collected from each peripheral blood donor were administered to at least four mice (the number of administered HSCs was 30,000–115,000 cells per animal). Two animals that received cells isolated from one donor were exposed before HSC administration (non-exposed human cells), and two mice that received cells isolated from the same donor were exposed after HSC administration (exposed human cells). The same exposure doses, 0.5, 1.0, and 1.5 Gy, were used (three donors per dose). Human and murine cells were enumerated three days and 14 days after exposure (one mouse with non-exposed human HSCs and another one with exposed cells for each timepoint).
The exposure was provided using the IGUR-1M radiobiological research gamma-unit (Quantum; Russia). The unit was equipped with the sources of 137Cs, the dose rate was 0.91 Gy/min, and the gamma field non-uniformity did not exceed 10%.
The number of murine CD45+ cells (stained with the PE-conjugated rat anti-CD45 monoclonal antibody, clone 30-F11; BD Pharmingen, USA), human CD45+ leukocytes (stained with the FITC-conjugated mouse anti-CD45 monoclonal antibody, clone HI30; Stem Cell Technologies, Canada), and CD45lowCD34+ human stem cells (stained with the APC-conjugated mouse anti-CD34 monoclonal antibody, clone 581; Stem Cell Technologies, Canada) in the bone marrow of mice was measured by flow cytometry. The Accuri C6 cytometer was used for measurement (BD Biosciences; USA). We calculated the number of cells per milliliter of suspension containing cells isolated from one bone.
The survival rate of exposed cells was calculated as a ratio of the number of exposed cells measured within three days and 14 days to the number of cells of the same donor in the control non-exposed mouse (for cord blood HSCs) or as a ratio of the number of exposed cells to the number of non-exposed cells of the same donor measured in the same timepoint after exposure (for peripheral blood HSCs). The HSC share was calculated as a percentage of the total number of human CD45low/CD45+ cells for each humanized animal. We also determined a coefficient that was calculated as a ratio of the HSC share on day 14 after exposure to the HSC share on day three after exposure (C14/3), since it was previously shown that this indicator was associated with the animal survival rate and cell repopulation in the bone marrow of non-humanized mice in in vivo studies [18].
Two experiments were conducted to assess the C14/3 coefficient prognostic properties in order to define the human HSC individual response to radiation exposure and the relationship between this indicator and radiosensitivity. The first experiment involved measuring the C14/3 coefficient for the fraction of murine CD117+ hematopoietic stem cells after exposure to the dose of 1 Gy in animals of two lines with different radiosensitivity: the radiosensitive NOD SCID line (LD50/30 = 3.5 Gy) and the relatively radioresistant C57Bl/6 line (LD50/30 = 6.0 Gy) [18].
In the second experiment, HSCs obtained from three cord blood donors were used for humanization in five humanized mice instead of three. These three additional humanized animals were administered the drug with a known radioprotective effect, mercaptoethylamine (cysteamine) at a dose of 200 mg/kg of body weight (Serva; USA), by intraperitoneal route 30 min before the exposure. The animals that received cells from two donors were exposed to a dose of 0.5 Gy, while the animals that received cells from the third donor were exposed to a dose of 1 Gy. We compared C14/3 coefficients in humanized mice not administered cysteamine and mice with increased radioresistance due to cysteamine.
The mean and standard error of the mean were calculated for the studied parameters. Regression analysis was performed using Microsoft Office Exсel (Microsoft; USA) to reveal the relationship between the studied indicators and the dose. Student's t-test was used to compare regression coefficients. The results were considered significant at the probability that the null hypothesis was true (p < 0.05).
RESULTS
After administration of the cord blood HSCs to mice with severe combined immunodeficiency (NOD SCID) human cells colonize the murine bone marrow and form a self-sustaining pool of HSCs and a pool of maturing cells, mostly lymphocytes/ granulocytes [17, 19]. This model makes it possible to study the radiation-induced apoptosis and repopulation of human stem cells, as well as their potential for maintaining the pool of maturing human cells in vivo in the humanized animal model.
Our experiments have shown that the humanized mice exposure results in the dose-dependent decrease in the survival rate of the CD45lowCD34+ human cells three days after exposure (fig. 1А). Exponential dose dependence is observed (R2 = 0.67; F = 38.65; p < 0.001).
On day 14 after exposure the number of HSCs in exposed mice increased. In different donors, it could exceed or fall below the baseline reference levels that corresponded to no exposure (fig. 1B). No significant correlation between the exposure dose and the decrease in the number of HSCs was observed on day 14 after exposure (R2 = 0.34; F = 4.01; p = 0.079 for linear relationship).
After exposure the share of stem cells among all human cells in the bone marrow of humanized mice decreased compared to baseline (25 ± 8%). There was a linear relationship between the dose within the range of 0.5–1.5 Gy and the C14/3 coefficient calculated as the ratio of the share of stem cells on day 14 after exposure to the share of stem cells on day three after exposure (fig. 2). This indicator decreased with increasing dose (R2 = 0.57; F = 13.26; p = 0.004). The indicator reflects the stem cells' survival rate, their regeneration 14 days after exposure, and ability to produce differentiating cells.
The experiment involving the use of radioprotective drug, cysteamine, showed that the humanized mice that were protected with radioprotector had a higher C14/3 coefficient than the non-protected mice exposed to the same dose (fig. 3). Thus, the increased coefficient indicates higher radioresistance.
The same pattern was revealed when comparing C14/3 coefficients in mice of the lines with different radiosensitivity (fig. 4). Thus, C14/3 coefficient in radiosensitive NOD SCID mice (LD50/30 = 3.5 Gy, 95% CI 3.4–3.8 Gy) exposed to a dose of 1 Gy was 0.23 ± 0.06, which represented a significant difference (t = 3.9; p = 0.003) from the C14/3 coefficient (0.98 ± 0.18) of the C57Bl/6 mice with normal radiosensitivity (LD50/30 = 6.0 Gy, 95% CI 5.8–6.2 Gy). This proves that the coefficient can reflect the differences in hematopoietic responses between mice with different radiosensitivity in vivo.
After administration of peripheral blood HSCs of adult humans, HSCs are unable to function in the bone marrow of mice for a long time, but can maintain the HSC pool and produce maturing cells for at least 14 days [17]. Just as in the model mice humanized with the cord blood HSCs, there is exponential relationship between the human HSC survival rate and the external gamma exposure dose on day three after exposure (R2 = 0.93; F = 211; p < 0.001; fig. 5А).
On day 14 after exposure, the number of HSCs increased and exceeded the control level (no exposure) in the animals exposed to a dose of 0.5 Gy; in the animals exposed to doses of 1.0 and 1.5 Gy, the number of cells remained low compared to the number of HSCs observed on day 14 in the non-exposed animals (fig. 5B). The linear model of the relationship between the dose and the number of HSCs relative to the non-exposed control 14 days after exposure was described (R2 = 0.65; F = 12.90; p = 0.009).
It is important to note that the models describing dose dependence of the cell survival rate that were obtained for the cord blood and peripheral blood HSCs were similar: no significant differences were revealed when comparing the coefficients (t = 1.18; p = 0.24) and the constant terms (t = 0.15; p = 0.88) of the equations that related the HSC survival rate and the dose on day three after exposure or when comparing the slope (t = 0.19; p = 0.85) and the constant terms (t = 0.34; p = 0.74) of the equations that related the relative cell number and the dose on day 14 after exposure.
Just like in the model mice humanized with the cord blood HSCs, C14/3 coefficient in the short-term model of mouse humanization with the peripheral blood HSCs was a function of dose (R2 = 0.45; F = 5.67; p = 0.048) and reflected individual characteristics of the HSC donors (fig. 6).
DISCUSSION
Assessment of individual radiosensitivity in terms of both severity of early tissue reactions and the risk of delayed exposure effects is necessary to identify the groups with high risk of adverse exposure effects (in case of high radiosensitivity) and to select staff (individuals with high radioresistance).
Individual response to radiation exposure can be measured at different levels of organization of the organism based on estimation of various final effects of exposure, such as death of the organism, cancer, non-cancerous disorders, tissue reactions after exposure, chromosomal aberrations, and molecular alterations [2, 3]. Not all studies succeed in determining the relationship between the molecular and cellular responses in vitro and the severity of tissue reactions to acute exposure [4, 20, 21].
It seems that clonogenic cell survival can be considered as a good predictor of radiosensitivity [4]. HSCs can be considered as a representational model for assessment of the hematopoietic system radiosensitivity. Obtaining humanized mice may be a promising method for assessment of the HSC individual response [8, 9], since these humanized mice may be considered as “avatars” reflecting the entire range of features of the cellular response inherent to the donor of cells. Such an approach is being developed within the framework of introducing personalized care for cancer patients and studying individual features of the immune system [22–25].
When human HSCs are transplanted to mice with severe combined immunodeficiency previously exposed to sublethal radiation dose, human cells can colonize the niches in the animal bone marrow that have become vacant after exposure and exist in the body of the mouse for a long time (2–12 months after administration). Moreover, HSCs not only maintain their pool of multipotent stem cells, but also give rise to the mature and functionally active blood cells, mostly myeloid cells.
The mice humanized by adminisration of the cord blood HSCs can be used for development of the acute radiation syndrome model, since these mice provide an opportunity to assess the effects of exposure on the HSC mortality, subsequent repopulation, and functional activity. Our study shows the the HSC survival rate in the short term after exposure (within three days) and the number of HSCs 14 days after exposure (during the period of restoring the population) depend on the dose in case of exposure to the doses of 0.5–1.5 Gy.
The C14/3 coefficient proposed by the authors that characterizes the change in the HSC share relative to other human CD45low/+ cells within 14 days after exposure is considered as an integrated indicator that reflects the HSC survival, subsequent restoration, and functional activity. The earlier studies show the relationship between this indicator and survival rates in mice of various lines, i.e. the association with radiosensitivity at the organism level [18]. The negative relationship between the C14/3 coefficient and the exposure dose has been revealed in the model mice humanized with the cord blood HSCs. This indicates the possibility of using this parameter for assessment of the severity of the tissue response to ionizing radiation. The experiments that involve comparison of these indicators in the organisms that obviously have different radiosensitivity (NOD SCID and C57Bl/6 mice, humanized mice administered or not administered radioprotector) have revealed unidirectional differences: the coefficient was higher in more radioresistant subjects. The association of higher C14/3 with the lower doses and higher radioresistance may be due to the more effective HSC repopulation after exposure, higher proliferative potential, and more effective restoration of the stem cell pool before triggering proliferation and maturation of the CD45+ cells.
When administering peripheral blood HSCs to mice with immunodeficiency, we have failed to obtain a long-lasting pool of stem cells in the animal bone marrow [17], however, simultaneous use of radiation exposure during transplantation of human cells and enumeration of HSCs and maturing hematopoietic cells within 14 days after transplantation is possible. The study shows that the dose dependence of the HSC survival rate and the HSC number 14 days after exposure is the same in the humanized mouse models with the sustained cord blood and peripheral blood HSCs. Therefore, the model obtained using peripheral blood HSCs that does not involve prolonged repopulation can be also used for simulation of acute radiation syndrome, as well as for assessment of the radiationinduced cell death and cell restoration. The calculated C14/3 coefficient has also shown negative correlation with the dose in the model obtained using peripheral blood HSCs.
It is important to note the fact that the HSC survival on day three after exposure in different donors of both cord blood and peripheral blood has shown much smaller individual differences than the number of HSCs that has raised within 14 days, the number of maturing CD45+ cells, and, consequently, the share of HSCs among all human cells and the С14/3 coefficient. It is clear that individual characteristics are less likely to affect the differences in the radiation-induced cell death and are more likely to affect the possibility of repair, subsequent restoration of the stem cell pool, and the HSC clonogenic activity.
The largest individual differences were observed in the humanized mice exposed to a dose of 0.5 Gy. Such exposure level may be recommended for assessment of individual HSC response that indicates individual radiosensitivity in the humanized mouse model obtained using peripheral blood HSCs.
CONCLUSIONS
The model humanized with human HSCs isolated from cord blood and peripheral blood can be used to assess the HSC response to radiation exposure in vivo. The C14/3 coefficient calculated as a ratio of the HSC share among all human CD45low/+ cells on day 14 after exposure to the same parameter on day three after exposure is the most effective integrated indicator that reflects the HSC radiation-induced death, restoration of the HSC number, and HSC functional activity. This coefficient can be used to estimate the human HSC individual response to radiation exposure.