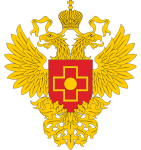
REVIEW
Role of radiology techniques and hybrid PET-MRI technique in the diagnosis of pharmacoresistant epilepsy
Federal Center of Brain Research and Neurotechnologies of the Federal Medical Biological Agency, Moscow, Russia
Correspondence should be addressed: Tatiana M. Rostovtseva
Ostovityanov, 1, bld. 10, 117513, Moscow, Russia; ur.liam@tavestvotsor
Funding: the study was performed as part of the research project “Developing Indications for the Use of Hybrid PET-MRI When Planning Surgery in Patients With Epilepsy”, code: 03.02.VY.
Author contribution: all authors contributed significantly to development of the concept, the study and manuscrit writing, they read and approved the final version of the article before publishing. The most significant contributions are distributed as follows: Dolgushin MB — study concept and plan; Rostovtseva TM, Duyunova AV, Nadelyaev RV Duyunova AV, Nadelyaev RV — data acquisition and analysis; Rostovtseva TM, Duyunova AV, Beregov MM — manuscrit writing.
Epilepsy is a disorder characterized by recurrent seizures caused by dysfunction of brain structures of different etiology and localization. The prevalence of epilepsy is 4–5 cases per 1000 population [1]. Despite the use of a wide variety of antiepileptic drugs, up to one quarter of patients (9–26%) remain resistant to antiepileptic therapy [2, 3].
Such invasive techniques, as resection surgery, endoscopic disconnection surgery, embolization, laser ablation, and stereotactic radiosurgery (Gamma Knife), are used for treatment of pharmacoresistant epilepsy. The effectiveness of surgical treatment is as high as 55–80%, it is directly dependent on the diagnosis accuracy [4, 5]. The goals of epilepsy surgery are to control seizures by resecting epileptogenic tissues and to minimize neuropsychological impairments and other neurological disorders by preserving important areas of the brain [6]. Accurate localization of epileptogenic zones and mapping of those against functionally significant cortical areas form an integral part of preoperative assessment of patients with pharmacoresistant epilepsy performed when planning surgery [7, 8]. According to multiple researchers, patients with epilepsy showing concordant EEG and MRI results recover fully after surgery in 30–90% of cases [9–11].
Conventional diagnostic algorithms for patients with epilepsy involve starting with video electroencephalography (EEG) monitoring, the noninvasive and rather accessible method. The use of scalp electrodes may be insufficient for accurate localization of foci in patients with the deep seated foci and rapidly propagating seizures [12].
MRI is the modality of choice for morphological confirmation of functional alterations detected by EEG that allows one to identify a whole range of abnormalities capable of causing epileptic seizures, such as tumors, vascular malformations, focal cortical dysplasia, mesial temporal sclerosis, brain matter alterations caused by injury or infarction, cortical migration disorders, and other conditions (fig. 1).
It should be noted that it is not possible to accurately localize epileptogenic foci using MRI and EEG in 20–50% of patients with pharmacoresistant epilepsy, or the MRI results are inconsistent with the data of video EEG monitoring and clinical features. In such cases, intracranial EEG may be used to clarify the epileptogenic focus localization [9, 10, 13], additional information may be also obtained by using functional MRI, MR spectroscopy or radionuclide diagnostics, SPECT and PET [3, 14]. The MR imaging-based morphometry (the method for quantitative assessment of volumes of various brain regions) may be used as a supporting techniques to clarify the epileptogenic focus localization and detect changes in the volumes of brain regions that are not directly related to the epileptogenic focus and are sometimes located in the contralateral hemisphere, which can be considered as a prognostic factor of the surgical treatment outcome [15].
Functional MRI can also be used for localization of the primary motor and sensory cortical areas, Wernicke's and Broca's areas, as well as the arcuate fasciculus, relative to the intended resection area in order to estimate the risk of neurologic deficit [16–18] (fig. 2).
Single photon emission computed tomography
The functional radionuclide diagnosis method, single photon emission computed tomography (SPECT), is widely used in assessing cerebral perfusion [19, 20]. Cerebral perfusion and metabolism are closely related in most physiological and pathological processes; cerebral blood flow (CBF) is often correlated with the neuronal activity. As compared with 18F-FDG PET used for assessment of the brain, SPECT that has lower spatial and higher temporal resolution makes it possible to detect a primary excitation focus. In the ictal phase, the increase in neuronal activity results in the increased metabolism and perfusion (CBF).
The regional cerebral blood flow measured using SPECT is considered to be an indirect marker of neuronal activity. Subtraction of ictal SPECT image from interictal image with subsequent co-registration to MRI using 99mTc-hexamethyl propylene amine oxime (HMPAO) or 99mTc-ethylene cysteine dimer (ECD) in parallel with EEG monitoring is the most sensitive and specific technique [21]. The technique involving sequential 99mTc-HMPAO SPECT and CT scanning within the same study is a more commonly used method.
Computational techniques enable estimation of differences between the SPECT perfusion indicators acquired in the ictal and interictal phases, and the results obtained are further co-registered to CT or MRI. Thus, SPECT allows one to clarify the presence of blood perfusion changes in the affected brain regions identified by neuroimaging or to reveal MR-negative regions showing abnormal perfusion [22–24] (fig. 3).
Xenon-133 (133Xe), the diffusing capacity of which allows one to measure cerebral blood flow (CBF) in mm/min per 100 g of tissue, is considered to be a reference radiopharmaceutical for estimation of perfusion. At the same time, 133Xe has a number of disadvantages, such as rapid clearance, short scan time and lower spatial resolution, that is why its clinical use is limited. In the past, 123I-amines were used along with 133Xe, however, the capability of redistibution imposes the same restrictions as the method involving the use of 133Xe. The above radioactive tracers now have mostly historical value.
Today, the most widely used radioactive tracers for perfusion imaging are technetium labeled compounds, the 99mTc-examethylpropyleneamine oxime (99mTc-HMPAO) and the technetium ethyl cysteinate dimer (99mTc-ECD). Redistibution is not typical for these radiopharmaceuticals, and their primary distribution is proportional to volumetric blood flow at the time of injection regardless of the blood flow fluctuations. This enables performing scanning within hours after the injection [22].
The recommended scan time for optimal signal-to-noise ratio is 30–90 min for 99mTc-HMPAO and 30–60 min for 99mTc-ECD. 99mTc-HMPAO and 99mTc-ECD have similar pharmacokinetic parameters and show comparable sensitivity and specificity when used for estimation of cerebral perfusion and localization of epileptogenic foci. The above radioactive tracers show differences in the mehanisms of absorption and dosimetry, as well as different patterns of distribution in brain matter: 99mTc-HMPAO is accumulated mainly in the cerebellum, while increased accumulation in primary visual cortex is typical for 99mTc-ECD. The features of the 99mTc-ECD pharmacokinetics are more selective fixation in brain tissues and more rapid urinary excretion allowing for administration of higher doses resulting in the higher quality of images compared to that obtained using 99mTc-HMPAO. According to some reports, 99mTc-ECD shows slightly higher sensitivity and specificity than 99mTc-HMPAO [23]. That is why it is advantageous to use the same radiopharmaceutical and the same scanning parameters when monitoring the dynamics [22]. The patient is recommended to stay in a stable environment, in a quiet, dimly-lit room at the time of the radioactive tracer injection and for several minutes before and after the injection to mitigate the impact of external stimuli on brain activation. When the patient is in a brightly lit room with his/her eyes open, the increased uptake of radiopharmaceutical in the visual cortex can be observed [23]. When performing interictal assessment, EEG monitoring is started 2 h before the injection and terminated not earlier than 15 min after the injection in order to avoid possible effects of epileptic seizure on the blood perfusion parameters: ictal hyperperfusion followed by hypoperfusion capable of affecting the entire cerebral hemisphere and even of contralateral spreading. Furthermore, the data of EEG monitoring are used to estimate bioelectric activity of the brain at the time of injection [20, 22]. When performing ictal assessment, the most accurate epileptogenic focus localization can be achieved when the radiopharmaceutical is injected no later than 20 s after the epileptic seizure. Assessment performed with administration of radiopharmaceutical after 45 s or later results in multiple ambiguous or even false results [25–27].
Ictal and interictal scans are visually assessed in accordance with the published guidelines [23, 28], in addition, quantitative indices are measured using ROI (region of interest), atlases of normal 99mTc-HMPAO and 99mTc-ECD distribution based on the data of healthy volunteers, voxel-based morphometry [23, 28]. After that image subtraction is performed: the areas of hyperperfusion revealed in interictal images are subtracted from ictal images.
The combined SPECT/MRI protocol (SISCOM, subtraction single photon emission CT co-registered to MRI) that implies overlaying the subtraction SPECT images on MRI scans, thereby allowing one to specify anatomic localization of alterations detected by SPECT and improve accuracy of the scan interpretation, is of special interest [29]. According to meta-analysis performed in 2016, co-registration of subtraction SPECT to MRI ensures positive prognostic value (PPV) of 56% when the data obtained using these techniques are concordant [30]. In addition to the diagnosis of MR-negative epileptogenic foci, the technique has found use in planning of partial resection of epileptogenic foci in patients with large zones of structural alterations. This can make it possible to achieve control over seizures. In addition, the technique can be used when planning re-surgery in patients with unacceptable results of previous surgical treatment [30, 31].
The literature data on comparison of the SPECT and [18F]-FDG diagnostic capabilities vary considerably depending on the study design, patient enrollment, and the expertise of medical personnel. According to retrospective studies performed in 2013, the SPECT sensitivity that reached 87% [32, 33] significantly exceeded the PET sensitivity (56%). Such high SPECT sensitivity values may be due to shorter time lag between the seizure and the radioactive tracer injection. The data of SPECT and PET can provide additional information and complement each other within the framework of multimodality approach to preoperative examination of patients with pharmacoresistant epilepsy [34].
Positron emission tomography
Another radionuclide brain imaging method, positron emission tomography (PET), is used to provide visualization and quantification of various cellular and biological processes, such as blood perfusion and metabolism, synthesis of proteins and DNA, receptor expression, etc. The detection feature of PET scanners is that images are formed entirely by the pairs of oppositely directed gamma rays, which results in higher spatial resolution compared to SPECT.
Standard preparation of a patient for PET study is conventionally performed in accordance with the European guidelines issued in 2021 [35]. Video EEG monitoring (10–20 electrode placement system) is performed within 2 h before intravenous administration of the radioactive tracer and throughout the period of the radiopharmaceutical uptake (at least 20 min) in order to ensure there are no seizures during the procedure. The patient should stay in a quiet dark room, in a comfortable position for at least 15 min before the radiopharmaceutical administration. The patient should be informed about the importance of maintaining a relaxed resting state before the procedure: to lay still with the eyes closed, not to talk, read or listen to music. If there is a need to use medical sedation, sedatives should be used as late as possible: at least 20 min after administration of radioactive tracer, several minutes before scanning. In case of static image acquisition procedure, scanning is started between 30 and 60 min after the radiopharmaceutical administration [36]. It is recommended to use the same scanning protocol, including the same scanning start time after administration of radiopharmaceutical when performing the follow-up assessment. When there is a need to level up the patient's motion artifacts or to perform quantitative analysis, the dynamic scanning can be carried out within the specified time interval; the optimal dynamic scanning start time is 60–90 min after the radiopharmaceutical injection [35].
There are a lot of radioactive tracers, however, 18F-fluorodeoxyglucose is most commonly used in clinical practice to identify epileptogenic foci. The use of 18F-fluorodeoxyglucose makes it possible to indirectly evaluate metabolism based on the quantitative changes in the glucose uptake. A rather long half-life (110 min) is one of the biggest advantages of 18F-FDG. Interictal PET is performed. This is due to the fact that sufficient 18F-FDG concentration in the brain is achieved 30–40 min after the injection, it reflects the summational metabolism of this period. Such a long radiopharmaceutical accumulation time makes it impossible to estimate transient neural processes, such as epileptic seizure that usually lasts one or few minutes [36].
Epileptogenic foci in patients with both temporal and extratemporal lobe epilepsy are associated with the areas of decreased glucose metabolism, which are usually larger that the foci themselves. The zone of 18F-FDG hypometabolism is likely to include the areas of initiation and propagation of excitation, that is why PET enables lateralization and only approximate localization of epileptogenic zones. However, it may be difficult to accurately define the area of the epileptic seizure origin using PET only, especially when the focus is small [37, 38].
In 10–43% of cases, additional hypometabolic zones, that are most often localized in the ipsilateral frontal lobe and are probably induced by the seizure, may be found in patients with epilepsy. Furthermore, the regional hypometabolism may be caused by a number of other factors not directly related to epilepsy: co-occurring structural alterations resulting from infarction, injury, infection, and neurodegenerative diseases, such as Alzheimer's disease. When interpreting the PET images, it should be borne in mind that reduced sensitivity of epileptogenic foci to 18F-FDG-PET can be observed in patients with focal-onset seizures prone to secondary generalization. That is why PET data interpretation should involve comparison with clinical data and EEG results [39, 40].
The 18F-FDG-PET results may be important for prediction of the disease course. Thus, identification of hypometabolic zones limited by the epileptogenic zone was associated with better surgical outcomes in patients with temporal lobe epilepsy, while no such correlation was found in patients with extratemporal lobe epilepsy [41, 42]. A mismatch between the hypometabolic zone localization according to PET, the epileptogenic zone revealed using EEG, and the clinical features may be a predictor of adverse surgical outcome. The combined analysis of PET and MRI data makes it possible to identify 81–95% of patients with beneficial surgical outcomes when the data obtained using these techniques are concordant [43]. The reasons for conflicting results have not been reliably established, however, there is a hypothesis that these discrepancies are due to the rapid spread of epileptic activity or the long history of epilepsy [43, 44]. The results of studies focused on assing sensitivity and specificity of positron emission tomography applied during preoperative planning in patients with pharmacoresistant epilepsy vary considerably. According to the meta-analysis conducted in 2007, positive prognostic value (PPV) reaches 72–89% depending on the study design [45].
PET. Antagonist of central GABA receptors
[1]1С-flumazenil (FMZ) is a specific reversible antagonist of the GABA receptor complex central benzodiazepine fragment. One of the papers reports reduced cortical 11С-FMZ binding in the epileptogenic foci of 85% of patients [46]. The zones with reduced cortical binding located at a distance from the epileptogenic focus have been also revealed in 55% of patients, which could complicate the diagnosis. The other studies show lower frequency of the areas of 11С-FMZ accumulation by epileptogenic zones compared to hypometabolic areas based on the 18F-FDG-PET data. This has a potential of more accurate epileptogenic foci localization [47, 48]. According to the metaanalysis conducted in 2021, the 11С-FMZ-PET sensitivity and specificity were 62% and 72%, these were comparable with the sensitivity and specificity of 18F-FDG-PET (66 and 71%, respectively). Moreover, higher sensitivity of 11С-FMZ in the subgroup of patients over 30 years of age having a long history of extratemporal lobe epilepsy was reported [48]. The results of the quantitative analysis involving the data of 18F-FMZ-PET and 18F-FDG-PET were consistent with the invasive EEG data in 86% and 71% of cases, respectively [49]. This allows us to consider these two techniques as a noninvasive diagnostic alternative.
PET. TSPO receptor ligands
Despite the fact that the first studies involving TSPO ligands, the neuroinflammation biomarkers, were conducted more than 20 years ago, new radioactive tracers have not yet found wide application in clinical practice. The results of a number of studies [50–52] confirm the increased accumulation of TSPO ligands in the epileptogenic substrate, which could be indicative of microglial activation and suggest the role of inflammation in the pathogenesis of epilepsy. A diffuse increase in the TSPO ligand uptake in brain matter, except for the cerebellum, within a week after status epilepticus has been reported in one of the studies. The authors suggest that duffuse accumulation of radiopharmaceutical may be a reflection of the “epileptic network”. After that the buildup of TSPO ligands was localized in the brain areas where epileptogenic foci were typically localized, amid continued low-intensity diffuse accumulation [53]. The correlation of TSPO expression with the surgical outcomes, drug resistance and behavioral disorders revealed in a number of experimental studies is of scientific interest and requires further investigation. TSPO ligands have potential as medications for assessment of the efficiency of new therapeutic strategies, as well as for prediction of the disease course and detection of drug resistance [54].
PET. Markers of metabolism and distribution of serotonin receptors
The results of pathomorphological assessment indicative of the increased serotonin synthesis in the epileptogenic cortex, including in patients with focal cortical dysplasia [55], necessitated the search for markers enabling its qualitative and quantitative assessment.α-11C-methyl-l-tryptophan (AMT), capable of tracing tryptophan metabolism through the serotonin and kynurenine pathway and detecting epipeptogenic foci in the interictal phase even in patients with tuberous sclerosis and cortical malformations, mostly IIB type, including those with no hypometabolic areas according to 18F-FDG-PET, is one such marker. The technique sensitivity is about 70%, and specificity is close to 100% [56]. These studies take on special importance in the diagnosis of the cortical tubers' epileptogenicity. Tuberous sclerosis is characterized by the presence of multifocal hypometabolic areas that significantly limits the use of 18F-FDG for estimation of each area epileptogenicity, although such estimation might have result in more selective surgical interventions, increased effectiveness of surgery and reduced surgical risks. According to the study conducted in 2013, the 11С-АМТ data were consistent with the data of ictal video EEG monitoring in 68 patients with type 1 and 2 tuberous sclerosis out of 95, and the more accurate localization of epileptogenic foci was achieved in 28 of patients. Moreover, the use of 11С-АМТ-PET made it possible to identify epileptogenic foci in 10 patients with no distinct epileptic foci according to the data of ictal EEG out of 17 [57]. According to other sources, sensitivity of the technique in the group of 12 patients with tuberous sclerosis was 17%, despite high specificity reaching 100%. This made it possible to identify epileptogenic foci in 17% of patients [58]. Little prior experience of using α-11C-methyl-l-tryptophan (AMT) PET is among other things due to short 11C half-life (about 20 min) and the consequent need to use cyclotron for AMT synthesis. That is why the subject of scientific inquiry is the search for ligands with longer half-life, including those based on [18F], the halflife of which is 110 min. One of such ligands used in scientific research, 18F-fluorobenzamidoethylpiperazine (18F-MPPF), is used to assess the distribution of 5‐hydroxytryptamine‐1A (5-HT1A) serotonin receptors allowing one to identify the areas of the synaptic serotonin release reduction due to astroglial activation [59]. According to one of the studies conducted in 2018, the 18F-MPPF accumulation was consistent with both 18F-FDG data and severity of epileptic seizures and behavioral disorders [60].
PET. Opioid receptor ligands
The following radioactive tracers are used to assess binding to various types of opioid receptors: 11C-carfentanil (11C-CFN), the µ opioid receptor agonist; 11C-N1'-methylnaltrindole (11C-MeNTI) that binds to — receptors; 11C-diprenorphine (11C-DPN), the nonselective opioid receptor agonist. As far back as in 1988–1991, there were reports about the changes in binding of µ and — opioid receptors in the temporal cortex ipsilateral to the epileptogenic focus during the postictal phase [61–63]. Lower frequency of areas showing the increased binding to opioid receptors compared to the areas with the decreased 18F-FDG uptake was noted. Later, in 2007, a hypothesis was put forward on the anticonvulsant effect of opioid peptides and the role of endogenous opioid system in the seizure control [64]. In 2023, the correlation of 11C-СFN binding to the µ opioid receptors with higher anxiety levels and depression was revealed in the group of patients with temporal love epilepsy. The authors assume that desensitization and inhibition of opioid receptors caused by seizures may represent a potential mechanism underlying the development of mood disorders often associated with epilepsy [65].
PET/MRI
The positron emission tomography and magnetic resonance imaging (PET/MRI) combined modality is a new hybrid technique that enables succesive or simultaneous assessment of structural and metabolic alterations in the brain within a single scan [66] (fig. 4). This hybrid, painless, minimally invasive technique shows high reproducibility, allows one to reduce the radiation dose, requires no careful and lengthy preparation for the study, and has a small number of contraindications and probable side effects [67]. The technique can be used for mapping of epileptogenic zones in patients with temporal lobe epilepsy [68–70] or epileptic zones located outside the temporal lobes [71]. PET/MRI has a number of advantages when used to assess patients with pharmacoresistant epilepsy. Among other things, the technique has a potential of increasing identification rate of focal cortical dysplasia (FCD) that is in some cases not easy to detect by MRI, although surgical treatment of such malformations can completely stop seizures or significantly reduce the severity of epilepsy. Assessment of the hybrid PET/MRI technique efficiency has shown better diagnostic outcomes and more accurate identification of epileptogenic foci (including in patients with refractory epilepsy) compared to the separate use of PET or MRI, and compared to PET-CT [71, 72].
Scientific studies published in the last few years used mathematical models (statistical and correlation analysis), allowing one to clarify the indications for PET/MRI aimed at noninvasive localization of epileptogenic zones, to confirm this hypothesis. According to the results of these studies, PET/MRI can be recommended to patients having discordant results of MRI and EEG along with no distinct epileptogenic foci, as well as to patients with multiple affected areas. The correlation analysis results have shown that PET/MRI can confirm the MR-positive epileptogenic foci and allows one to distinguish patients having indications for surgical treatment from inoperable patients. The post-processing techniques, such as calculation of the asymmetry index using the brain atlases and statistical parametric mapping (SPM) analysis, can provide quantitative confirmation of visual PET/MRI scan assessment along with additional information required for the treatment tactics selection [73]. The method for automated analysis of the PET data interhemispheric metabolic asymmetry performed after the automated anatomical symmetrization co-registered to MRI (the PASCOM protocol) is independent from the PET atlases of healthy volunteers, less limited, it enables more accurate lateralization and localization of the epileptogenic zone [74].
Simultaneous fMRI-EEG acquisition represents an alternative noninvasive epileptogenic zone imaging method. According to the studies piblished in 2012, positive prognostic value of detecting the epileptiform activity and the BOLD response changes within 2 cm from the epileptogenic zone was as high as 78%, while negative prognostic value was as high as 81% [74–77]. The technique involving simultaneous fMRI-EEG acquisition also makes it possible to discern changes in the BOLD response at the seizure onset, during the propagation of excitation, and in the pre-ictal phase [78].
CONCLUSION
Essential steps of preoperative assessment of patients with pharmacoresistant epilepsy are represented by video EEG monitoring (for ictal EEG recording and analysis of epileptiform patterns by neurophysiologist) and MRI (for detection of morphological changes in the brain). The surgical treatment outcome is directly dependent on the accuracy of the epileptogenic substrate localization by the diagnostic technique. At the same time, the MRI and video EEG monitoring results are not always concordant, in some cases MRI and EEG are insufficient for both accurate localization of epileptogenic foci and distinguishing between operable and inoperable epileptogenic foci.
Despite the significant number of papers, the data of which show benefits of using single photon emission and positron emission tomohraphy, as well as hybrid PET/MRI modality, when selecting candidates for surgery, the optimal plan for preoperative assessment of patients with pharmacoresistant epilepsy remains the subject of scientific debate. The algorithm for selection of the radioisotope technique or the combination of such techniques in patients with various disorders resulting in pharmacoresistant epilepsy requires further investigation.
Currently, the SPECT/CT technique enables quantitative estimation of the differences in blood perfusion changes observed in the affected brain regions in the inctal and interictal phases with subsequent coregistration to MRI for clarification of the epileptogenic nature of the detected structural changes in the brain or localization of MR-negative regions showing abnormal blood perfusion.
PET is used maily in the interictal phase, that is why a less thorough preparation compared to SPECT/CT is required. The technique enables lateralization and localization of the area of the excitation initiation and propagation. The combined analysis of PET, MRI and EEG data may be effective in evaluating the epileptogenic zone resectability and predicting the surgical outcome. Further studies of the ligands binding to the translocator protein (TSPO) receptors, opiod receptors and serotonin metabolism markers are of great scientific interest. The use of such ligands has a potential of improving the epileptogenic substrate detection specificity and the surgical treatment selectivity, especially in patients with focal cortical dysplasia and tuberous sclerosis, and can change perceptions about the pathogenetic mechanism of epilepsy and mood disorders often co-existing with epilepsy.
The hybrid PET/MRI technique, including involving the use of the most accessible 18F-FDG molecule, has a potential of significant contribution to the development of the algorithm of preoperative assessment of patients with pharmacoresistant epilepsy and will make it possible to clarify the presence of epileptogenic zones in patients with dubious MRI results, small foci or ambiguous metabolic patterns. Introduction of correlation analysis into clinical practice is one promising direction of the method development, the obtained quantitative data can be useful in determining the possibility of surgical treatment in patients with pharmacoresistant epilepsy.